- 1Research Center on Public Health, University of Milan Bicocca, Monza, Italy
- 2Laboratory of Public Health, Auxologico Research Hospital—IRCCS, Milan, Italy
- 3Dipartimento di Anestesia, Rianimazione ed Emergenza Urgenza, Fondazione IRCCS Ca’ Granda Ospedale Maggiore Policlinico, Milano, Italy
- 4Institute for Risk Assessment Sciences (IRAS), Utrecht University, Utrecht, Netherlands
- 5Epidemiology Unit, Department of Prevention, Central Tuscany Local Health Authority, Florence, Italy
- 6Health Activities and Epidemiological Observatory Department, Health Authority Sicily Region, Parlemo, Italy
- 7Clinical Pathology Complex Hospital Unit, Health Authority Trapani Province, Trapani, Italy
- 8Department of Cardiovascular, Endocrine-Metabolic Disease and Aging, National Institute of Health (ISS), Rome, Italy
- 9Laboratory for Industrial and Applied Mathematics (LIAM), Department of Mathematics and Statistics, Faculty of Science, York University, Toronto, ON, Canada
- 10Department of Hygiene and Health Prevention and Complex Operative Unit Environmental Health and Innovative Projects, Health Protection Agency, Pavia, Italy
- 11Department of Clinical Sciences and Community Health, University of Milan, Milan, Italy
- 12Epidemiology Unit, Fondazione IRCCS Ca’ Granda Ospedale Maggiore Policlinico, Milan, Italy
- 13Servizio Epidemiologico Aziendale, Agenzia di Tutela della Salute di Bergamo, Bergamo, Italy
- 14Neurology, Public Health and Disability Unit, Carlo Besta Neurological Institute, Fondazione IRCCS Istituto Neurologico Carlo Besta, Milan, Italy
- 15Department of Public Health, University of Naples Federico II, Naples, Italy
- 16Department of Occupational and Environmental Medicine Epidemiology and Hygiene, Italian National Workers Compensation Authority (IIL), Monteporzio Catone, Italy
- 17International Network of Public Health and Environment Tracking (INPHET), Milan, Italy
- 18Department of Epidemiology, Lazio Region Health Authority (ASL RM1), Rome, Italy
- 19Occupational Health Unit, Department of Medical and Surgical Sciences, University of Bologna, Bologna, Italy
- 20Department of Juridical and Economic Studies, Faculty of Law, Sapienza University of Rome, Rome, Italy
- 21Department of Experimental Medicine, University of Campania Luigi Vanvitelli, Naples, Italy
- 22Department of Translational Medicine, University of Eastern Piedmont, Novara, Italy
- 23Department of General Surgery and Medical-Surgical Specialties, University of Catania, Catania, Italy
- 24Department of Oncology, Mario Negri Pharmacological Research Institute (IRCCS), Milano, Italy
- 25Department of Woman and Child Health and Public Health, Fondazione Policlinico Universitario A. Gemelli IRCCS (Agostino Gemelli University Polyclinic IRCCS), Rome, Italy
- 26Global Health Research Institute, Università Cattolica del Sacro Cuore (Catholic University of Sacred Heart), Rome, Italy
- 27Institute for Cancer Research, Prevention and Clinical Network (ISPRO), Florence, Italy
- 28Department of Medicine, University of Udine, Udine, Italy
- 29Department of Mental Health, Healthcare Agency “Friuli Occidentale”, Pordenone, Italy
- 30Department of Pharmacological and Biomolecular Sciences, University of Milan, Milan, Italy
- 31MultiMedica, IRCCS, Sesto San Giovanni, Italy
- 32Department of Public Health and Infectious Diseases, Sapienza University of Rome, Rome, Italy
- 33Institute of Neuroscience, National Research Council (CNR), Pisa, Italy
- 34IRCCS Istituto Ortopedico Galeazzi (Galeazzi Orthopedic Institute IRCCS), University of Milan, Milan, Italy
- 35Department of Dermatology, Case Western Reserve University, Cleveland, OH, United States
- 36Department of Biomedical and Neuromotor Sciences, University of Bologna, Bologna, Italy
- 37Department of Environmental Health Sciences, Mario Negri Institute for Pharmacological Research, Milan, Italy
- 38Institute for Health Metrics and Evaluation, University of Washington, Seattle, WA, United States
- 39Department of Health Metrics Sciences, School of Medicine, University of Washington, Seattle, WA, United States
- 40Department of Clinical and Experimental Medicine, University of Catania, Catania, Italy
- 41Department of Biomedical and Dental Sciences and Morphofunctional Imaging, Messina University, Messina, Italy
- 42Clinical Research Department, IRCCS Fondazione Don Carlo Gnocchi, Milan, Italy
- 43Department of Neurology, IRCCS Humanitas Research Hospital, Milan, Italy
- 44Istituto di Ricerche Farmacologiche Mario Negri IRCCS, Bergamo, Italy
- 45Daccude, Todi, Italy
- 46Department of Law, Economics, Management and Quantitative Methods, University of Sannio, Benevento, Italy
- 47WSB University in Gdańsk, Gdańsk, Poland
- 48Department of Medical, Surgical Sciences and Advanced Technologies, University of Catania, Catania, Italy
- 49Clinical Epidemiology and Public Health Research Unit, Burlo Garofolo Institute for Maternal and Child Health, Trieste, Italy
Objectives: We explored temporal variations in disease burden of ambient particulate matter 2.5 μm or less in diameter (PM2.5) and ozone in Italy using estimates from the Global Burden of Disease Study 2019.
Methods: We compared temporal changes and percent variations (95% Uncertainty Intervals [95% UI]) in rates of disability adjusted life years (DALYs), years of life lost, years lived with disability and mortality from 1990 to 2019, and variations in pollutant-attributable burden with those in the overall burden of each PM2.5- and ozone-related disease.
Results: In 2019, 467,000 DALYs (95% UI: 371,000, 570,000) were attributable to PM2.5 and 39,600 (95% UI: 18,300, 61,500) to ozone. The crude DALY rate attributable to PM2.5 decreased by 47.9% (95% UI: 10.3, 65.4) from 1990 to 2019. For ozone, it declined by 37.0% (95% UI: 28.9, 44.5) during 1990–2010, but it increased by 44.8% (95% UI: 35.5, 56.3) during 2010–2019. Age-standardized rates declined more than crude ones.
Conclusion: In Italy, the burden of ambient PM2.5 (but not of ozone) significantly decreased, even in concurrence with population ageing. Results suggest a positive impact of air quality regulations, fostering further regulatory efforts.
Introduction
Air pollution represents a paradigm of risk factor associated to relatively modest increases in the individual risk, but to substantial disease burden at the population level [1]. The World Health Organization (WHO) recognized air pollution as a health risk factor in 1958 [2], with effects ranging from subclinical lesions to premature death [3, 4]. Although directives have been thereafter issued, it remains a substantial public health concern owed to its mortality and disability burden [5–7]. In 2008, the European Union (EU) introduced the “Air Quality Directive”, fixing target values for long-term concentrations of air pollutants [4]. As a plausible effect, a reduction of 22% in annual mean concentrations of PM2.5 was observed across Europe from 2009 to 2018. Conversely, an increase in ozone concentrations was registered over the same period, owed to increasing in precursors emission and changes in regional climate characteristics [1, 8]. The 2021 WHO Global Air Quality Guidelines updated air quality levels, focusing on six pollutants: particulate matter with diameter equal or smaller than 2.5 and 10 µm (PM2.5 and PM10), ozone, nitrogen dioxide, sulfur dioxide and carbon monoxide [3]. In this frame, it is compelling to understand the health impact of regulation-driven modifications in pollutant concentrations.
The Global Burden of Diseases (GBD) Study 2019 graded air pollution as fourth-ranking risk factor for mortality and disability-adjusted life years (DALYs) globally, accounting for 85.6 deaths (95% Uncertainty Interval [95% UI]: 75.7, 96.1) and 2791.1 DALYs (95% UI: 2468.8, 3141.4) per 100,000 people [7]. These estimates quantify health impacts attributable to exposure to ambient and household PM2.5 and ambient ozone, and account for morbidity and mortality of selected diseases associated with pollution: chronic obstructive pulmonary disease (COPD), lower respiratory infections (LRI), ischemic heart disease (IHD), stroke, tracheal bronchus and lung (TBL) cancer, type 2 diabetes mellitus (T2DM), premature birth and decreased birthweight [1, 7].
Specific evidences from the GBD Study underlined that deaths and DALYs due to PM2.5 long-term exposure globally increased from 1990 to 2015. Meanwhile, an increment of ozone-attributable COPD deaths was observed [9]. These trends might result from several phenomena that add up to the variation in pollutants concentrations, such as changes in the population age structure or variations in mortality and morbidity rates [8–13].
Using GBD estimates, we investigated temporal variations in disease burden from long-term exposure to ambient PM2.5 and ozone from 1990 to 2019 in Italy, with the aim of disentangling the effect of the reduction in air pollution concentration from demographic (population ageing) and epidemiologic (mortality and morbidity rates) population dynamics.
Methods
Overview
The GBD Study provides comprehensive global estimates of disease burden, such as incidence, prevalence, mortality, years of life lost (YLLs), years lived with disability (YLDs), healthy life expectancy (HALE) and DALYs, for 204 countries and territories [7]. It also applies a comprehensive and standardized method to identify risk factors and risk-outcome associations by disease cause. Risk factors are organized in five hierarchical nested levels; risk-outcome pairs are assessed for inclusion based on availability and strength of the evidence for a causal association, and on the feasibility of developing complete estimates of exposure levels [7, 14].
The burden attributable to a risk factor is estimated for each risk–outcome pair based on the overall estimate of the outcome burden, spatial and temporal exposure estimates for the risk factor, the theoretical minimum risk exposure level (TMREL) and the relative risk, or dose-response function, describing the association between risk factor and outcome [15].
Here, we focus on the burden of long-term exposure to ambient PM2.5 (GBD level four risk factor) and ozone (GBD level three risk factor) pollution. Detailed descriptions of data sources, metrics and methods are available elsewhere [7]. The study is compliant with the Guidelines for Accurate and Transparent Health Estimates Reporting [16].
Ambient PM2.5 Exposure and Associated Risk-Outcome Pairs
Input data used by GBD 2019 to estimate population-weighted exposure to ambient PM2.5 included ground measurements, satellite-based estimates, chemical transport model simulations, and population estimates. GBD 2019 data sources for ground measurements of PM2.5 consisted of updated measurements from GBD 2017 and additional measurements provided by the WHO Global Ambient Air Quality Database in 2018. A hierarchy of conversion factors (PM2.5/PM10 ratios) was used to obtain PM2.5 values for locations containing only measurements of PM10. The satellite-based estimates combined information on aerosol optical depth retrievals from multiple satellites, chemical transport model simulations and information on land use, and were available on a spatial resolution of 0.10 × 0.10 (which corresponds to 11 × 11 km at the equator) [17]. Population estimates were derived from the Gridded Population of the World database for the years 1990, 1995 (3rd version), and 2000, 2005, 2010, 2015, 2020 (4th version), and natural spline interpolation was used to calculate population estimates in intermediate years. PM2.5 estimates in Italy were calculated using a Bayesian hierarchical calibration model, thus combining information from satellite retrievals, chemical transport model simulations and population estimates calibrated with ground monitor PM2.5 estimates. The TMREL for ambient particulate matter was estimated on a uniform distribution with lower and upper bounds corresponding to 2.4 and 5.9 μg/m3 based on the average of the minimum and 5th percentiles of exposure distributions to air pollution in the cohort studies used to produce the GBD estimates.
Risk-attributable disease burden for ambient PM2.5 was computed for risk-outcome pairs validated for inclusion using the scientific literature. Such validation procedure identified the following GBD level three outcomes (causes): IHD, stroke, COPD, T2DM, LRI, TBL cancer, and neonatal disorders [7, 14].
In order to ascertain the shape of the dose-response relationship for each outcome, the GBD 2019 adopted the Meta-Regression-Bayesian Regularized Trimmed (MR-BRT) strategy, with input data from studies assessing the effect of PM2.5 ambient and household pollution [6, 16, 18–20].
Ambient Ozone Exposure and Associated Risk-Outcome Pairs
GBD 2019 definition of ozone ambient air pollution is the highest seasonal (6 months) average of 8-h daily maximum ozone concentrations measured as parts per billion (ppb), for each 0.10 × 0.10 grid cell on a global scale. Ozone ground measurements obtained from the Tropospheric Ozone Assessment Report and continent-specific chemical transport models provided by the Chemistry-Climate Model Initiative were combined in a single geo-statistical modelling tool, the Bayesian Maximum Entropy, to estimate the exposure to ambient ozone pollution globally for the period 1990–2017. Subsequently, a log-linear model was run to extrapolate ambient ozone pollution exposure for the years 2018 and 2019. The TMREL for ambient ozone air pollution ranged between 29.1 and 35.7 ppb [7, 10].
In GBD Study 2019, estimation of ambient ozone pollution attributable disease burden is based on a literature review exploring long-term ozone exposure and COPD mortality including five cohort studies, followed by MR-BRT risk splines estimation [7, 14].
Analysis
We used GBD estimates for population-weighted exposure, population age structure, deaths, DALYs, YLLs and YLDs attributable to ambient PM2.5 and ozone in Italy from 1990 to 2019 [21, 22]. We also obtained GBD estimate for the global population age structure in 2019. For ozone, estimated DALYs corresponded to YLLs, as YLDs were always null, since literature only supported the association with COPD mortality. We downloaded the same measures also for the overall burden of each disease associated with PM2.5 and ozone. Crude and age-standardized rates per 100,000 person-years with 95% UI were considered for all estimates. Age-standardized rates were computed through a direct standardization, using GBD 2019 World Standard Population as a reference [23].
The temporal evolution of the burden attributed to ambient PM2.5 and ozone pollution from 1990 to 2019 was analyzed both as annual rates and percent variation (95% UI) in rates from 1990 to 2019, 1990 to 2010, 2010 to 2019. This analysis was stratified by age, sex and cause-specific disease burden.
To highlight the contribution of demographic and epidemiologic dynamics in the variation of disease burden attributable to ambient pollution, we first compared time changes of crude and age-standardized rates attributable to ambient PM2.5 and ozone pollution, as the discrepancy in the temporal evolution of these two measures is related to population aging. Crude rates depict the real estimated variation based on the age-structure of the Italian population, while age-standardized ones report the expected variation if the population maintained a fixed age-structure equal to that of the global one. Therefore, the decrease observed for age-standardized rates can be interpreted as the expected decrease in the absence of population ageing. Then, we compared time changes in overall diseases burden to that attributable to PM2.5 and ozone, through age-standardized rates. These comparisons were carried out both graphically and on percent variations (95% UI) over 1990–2010 and 2010–2019 periods.
Results
The Burden of Ambient Air Pollution in 2019
In 2019, in Italy, ambient PM2.5 pollution accounted for 24,700 deaths (95% UI: 19,200, 30,000) and 467,000 DALYs (95% UI: 371,000, 570,000), corresponding to 3.8% (95% UI: 3.0, 4.7) of total deaths, and 2.6% (95% UI: 2.0, 3.2) of total DALYs. The burden attributable to ambient ozone pollution was lower, with 3,490 deaths (95% UI: 1,600, 5,390) and 39,600 DALYs (95% UI: 18,300, 61,500), corresponding to 0.5% (95% UI: 0.3, 0.8) and 0.2% (95% UI: 0.1, 0.3), respectively.
Crude DALY rates per 100,000 inhabitants due to ambient PM2.5 amounted to 773.5 (95% UI: 614.5, 945.0). The burden was higher among males (924.5, 95% UI: 734.2, 1122.2) than in females (630.5, 95% UI: 492.3, 781.1). Children aged up to 5 years had higher DALY rates (149.1, 95% UI: 99.9, 206.6) than those aged 5 to 14 (0.9, 95% UI: 0.5, 1.4). Within the following age classes, an increasing trend emerged, reaching a maximum of 1944.8 (95% UI: 1511.9, 2391.0) among people aged 75 or more. The top three conditions associated to PM2.5 exposure in relation to DALY rate per 100,000 were IHD, T2DM and stroke (Table 1, Supplementary Tables S1–S3).
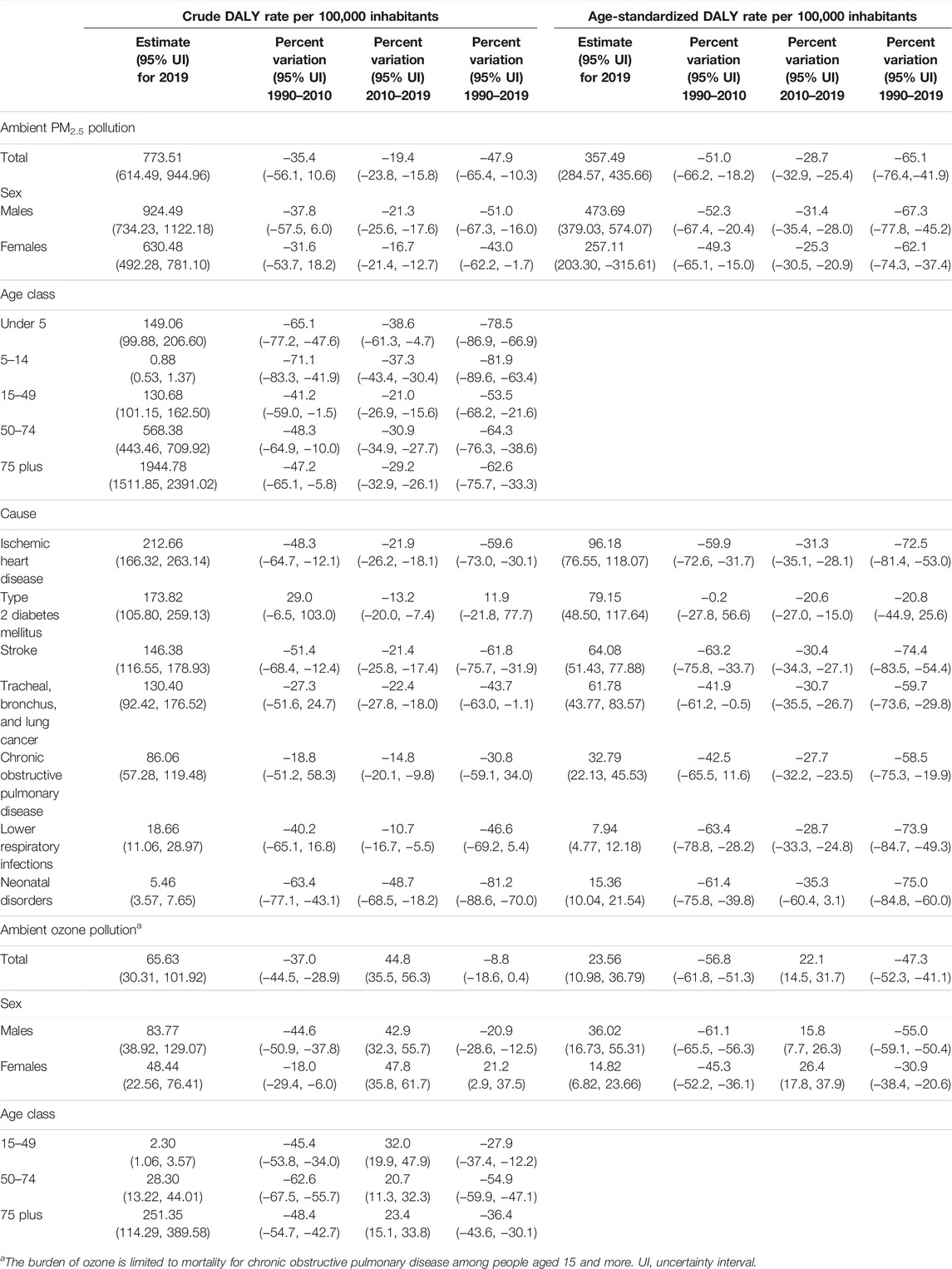
Table 1. Crude and age-standardized rates of disability-adjusted life years (DALYs) due to ambient particulate matter and ozone pollution in 2019. Temporal variation from 1990 to 2010, 2010 to 2019 and from 1990 to 2019 (Global Burden of Disease Study, Italy, 1990–2019).
As for ambient ozone pollution, the total crude DALY rate per 100,000 inhabitants was 65.6 (95% UI: 30.3, 101.9), (Table 1). The burden was higher among males (83.8, 95% UI: 38.9, 129.1) than in females (48.4, 95% UI: 22.6, 76.4), and it increased with age, starting from 2.3 (95% UI: 1.1, 3.6) among people aged 15–49 and rising to 251.4 (95% UI: 114.3, 389.6) among those aged 75 or more (Table 1).
Age-standardized DALY rates were 357.5 (95% UI: 284.6, 435.7) and 23.6 (95% UI: 11.0, 36.8), respectively for ambient PM2.5 and ozone. The difference between males and females persisted: estimated DALY rates were respectively 473.7 (95% UI: 379.0, 574.1) and 257.1 (95% UI: 203.3, 315.6) for PM2.5, and 36.0 (95% UI: 16.7, 55.3) and 14.8 (95% UI: 6.8, 23.7) for ozone. Furthermore, when stratifying by condition associated with PM2.5, the pollutant burden confirmed to be highest for IHD and T2DM (Table 1).
A thorough description of mortality, YLLs and YLDs attributable to PM2.5 and ozone is reported in Supplementary Tables S1–S3. The overall crude mortality rate was 40.9 (95% UI: 31.8, 49.8) per 100,000 inhabitants, while the age-standardized one was 14.8 (95% UI: 11.8, 17.9) (Supplementary Table S1). YLLs outweighed YLDs, with age-standardized rates of respectively 269.8 (95% UI: 220.0, 320.5) and 87.7 (95% UI: 56.1, 125.4) (Supplementary Tables S2, S3). Differences between sexes were also confirmed for mortality and YLL rates. The distribution among age classes mirrored that observed for DALYs for all other burden measures. Notably, YLDs exceeded YLLs for T2DM, and they represented a consistent share of the total DALYs for COPD and stroke.
Temporal Trends
The population-weighted average concentration of PM2.5 decreased from 26.9 μg/m3 in 1990 to 16.1 μg/m3 in 2019 (Supplementary Figure S1). This was mirrored by a clear decreasing trend in the burden from 1990 to 2019 in terms of crude and age-standardized mortality, DALY and YLL rates, while YLD rates showed a rather stable trend (Figure 1). However, variations in crude rates were significantly lower than those observed for age-standardized rate. Crude DALY, mortality and YLL rates decreased by 47.9% (95% UI: 10.3, 65.4), 41.9% (95% UI: 3.2, 62.1) and 55.4% (95% UI: 22.6, 70.5), while the corresponding age-standardized rates decreased by 65.1% (95% UI: 41.9, 76.4), 67.5% (95% UI: 43.1, 78.8) and 70.5% (95% UI: 51.1, 80.1) (Table 1; Supplementary Tables S1–S3). When comparing the periods 1990–2010 and 2010–2019, significant differences in crude and age-standardized rates variations persisted only within the second period (Table 1; Figure 2). Focusing on DALYs, crude rates declined by 19.4% (95% UI: 15.8, 23.8), while the age-standardized ones decreased by 28.7% (95% UI: 25.4, 32.9). Variations observed for mortality and YLLs were similar to those of DALYs while YLDs showed slightly milder declines (Table 1; Supplementary Tables S1–S3; Figure 2). Focusing on age-specific rates, the decrease appeared to be slightly higher among people aged less than 14 for all the measures, while the minimum decrease in terms of DALYs was observed for people aged 15–49, due to a 29.5% (95% UI: 25.7, 33.6) decrease in YLL rates that is partially counterbalanced by a 6.8% (95% UI: −5.1, 18.3) increase in YLD rates (Table 1; Supplementary Tables S1–S3). Crude 2010–2019 DALY rates decrease ranged from 10.7% (95% UI: 5.5, 16.7) for LRI to 48.7% (95% UI: 18.2, 68.5) for neonatal disorders, but when considering age-standardized rates, all decreases were close to 30%, with the exception of T2DM (20.6%, 95% UI: 15.0, 27.0). A similar consideration applied to YLLs, while differences were even more pronounced when considering mortality (Figure 2 and Supplementary Tables S1–S3).
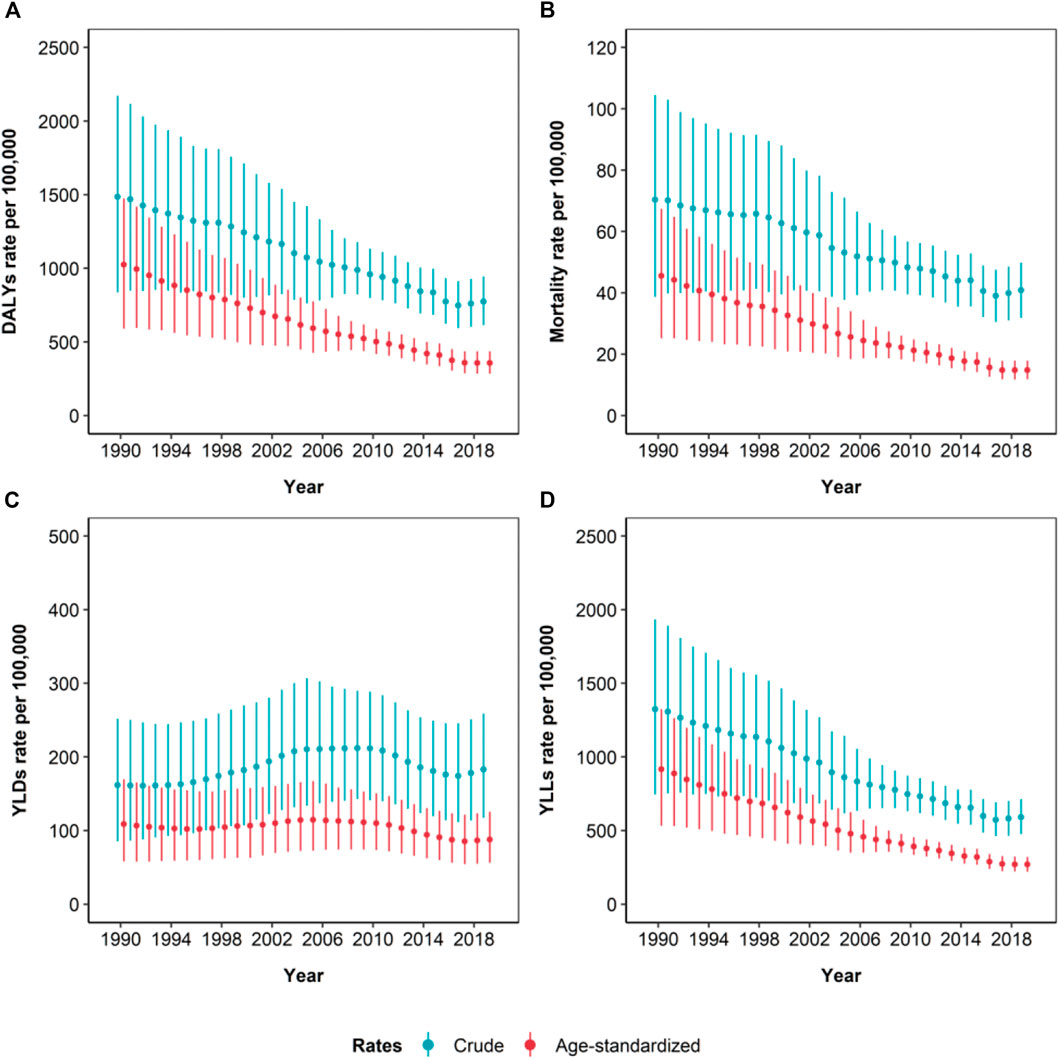
Figure 1. Time-series of the estimated crude and age-standardized rates (per 100,000 inhabitants) of disability adjusted life years (DALYs) (A), mortality (B), years lived in disability (YLDs) (C), and years of life lost (YLLs) (D), due to ambient particulate matter pollution, from 1990 to 2019. Whiskers represent 95% Uncertainty Intervals (Global Burden of Disease Study, Italy, 1990–2019).
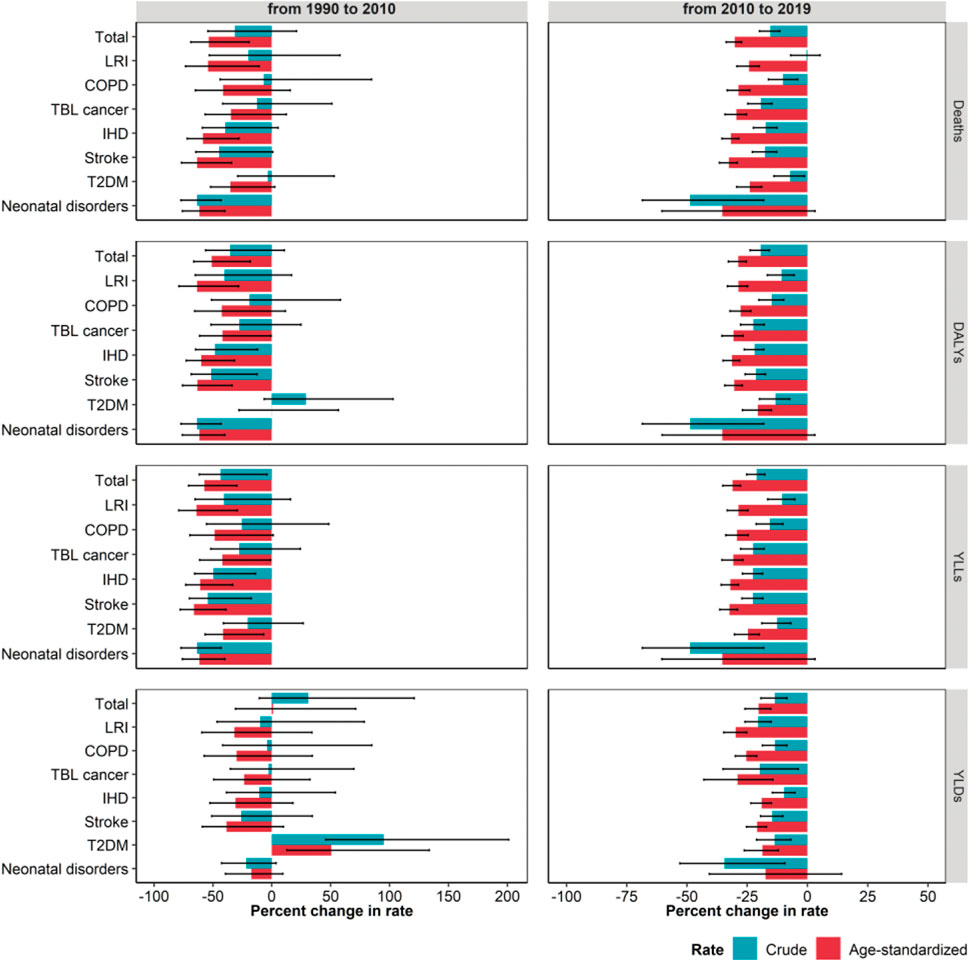
Figure 2. Estimated percent change in crude and age-standardized rates (per 100,000 inhabitants) of mortality (deaths), disability adjusted life years (DALYs), years of life lost (YLLs) and years lived in disability (YLDs) due to ambient particulate matter pollution, from 1990 to 2010 and from 2010 to 2019, stratified by cause. Whiskers represent 95% Uncertainty Intervals. LRI, Lower respiratory infections; COPD, Chronic obstructive pulmonary disease; TBL, Tracheal, bronchus and lung; IHD, Ischemic heart disease; T2DM, Type 2 diabetes mellitus (Global Burden of Disease Study, Italy, 1990–2019).
Ambient ozone pollution burden described no clear decline during the whole period 1990–2019. Indeed, crude DALY and mortality rates declined by 37.0% (95% UI: 28.9, 44.5) and 20.6% (95% UI: 11.5, 30.4) during 1990–2010, but they increased by 44.8% (95% UI: 35.5, 56.3) and 52.6% (95% UI: 42.9, 64.8) during the following period. A similar trend was observed for age-standardized rates (Table 1; Supplementary Table S1; Figure 3).
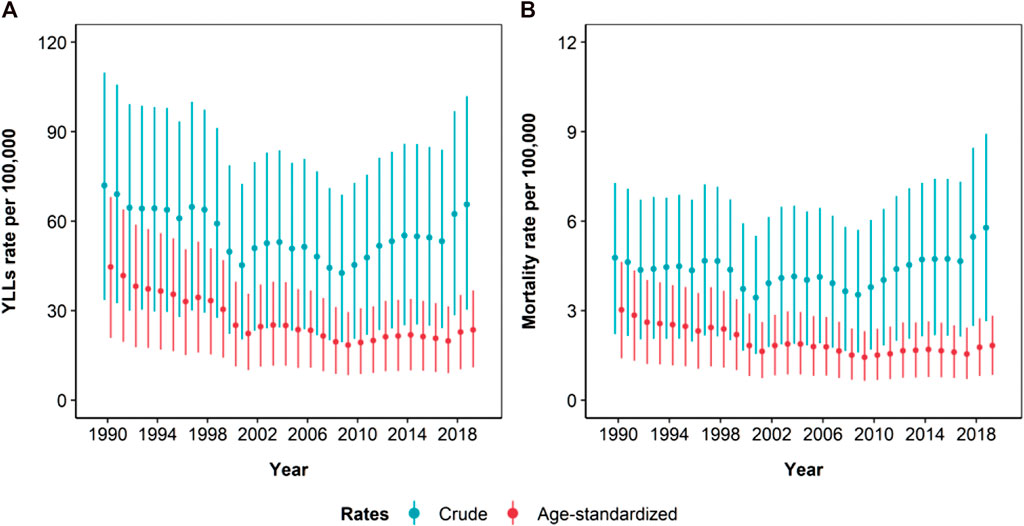
Figure 3. Time-series of the estimated crude and age-standardized rates (per 100,000 inhabitants) of years of life lost (YLLs) (A)* and mortality (B) due to ambient ozone pollution, from 1990 to 2019. Whiskers represent 95% Uncertainty Intervals (Global Burden of Disease Study, Italy, 1990–2019). *YLLs account for the whole DALYs amount.
The decrease observed from 1990 to 2010 was more pronounced for age-standardized rates, while in the following period the increase was higher for crude rates (Figure 4).
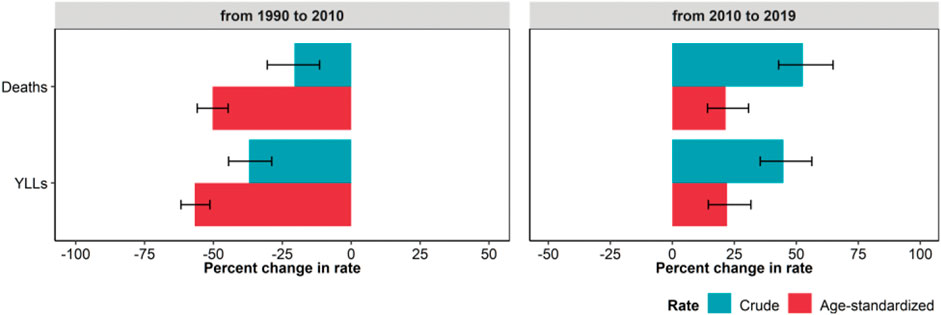
Figure 4. Estimated percent change in crude and age-standardized rates (per 100,000 inhabitants) of mortality (deaths), and years of life lost (YLLs*) due to ambient ozone pollution, from 1990 to 2010 and from 2010 to 2019. Whiskers represent 95% Uncertainty Intervals (Global Burden of Disease Study, Italy, 1990–2019). *The burden of ozone is limited to chronic obstructive pulmonary disease mortality, therefore YLLs account for the whole DALYs amount.
Variations in the burden reflected trends in the population-weighted average ozone concentration, that oscillated from 56.6 ppb in 1990 to 48.7 ppb in 2010 to 54.1 ppb in 2019 (Supplementary Figure S1).
Understanding the Contribution of Exposure Variation
For all diseases, measures referred to the overall burden but YLDs displayed a significant decline during the study period, especially during 1990–2010, with the exception of T2DM (Supplementary Figures S2–S8). Indeed, during that period, reductions in age-standardized DALY rates ranged from 27.7% for COPD (95% UI: 23.0, 30.1) to 54.3% for stroke (95% UI: 52.5, 56.8). YLL and mortality rates showed similar declines, while YLD rates showed moderate declines, and two diseases, namely neonatal and T2DM, faced an increase of respectively 9.1% (95% UI: 0.5, 18.7) and 59.3% (95% UI: 48.5, 71.6) (Figure 5; Supplementary Table S4). For DALYs, YLLs and mortality, percent reductions were similar for the overall burden and for the burden attributable to ambient PM2.5, while the percent reduction in ozone attributable burden was significantly higher than the overall burden of COPD (Figure 5).
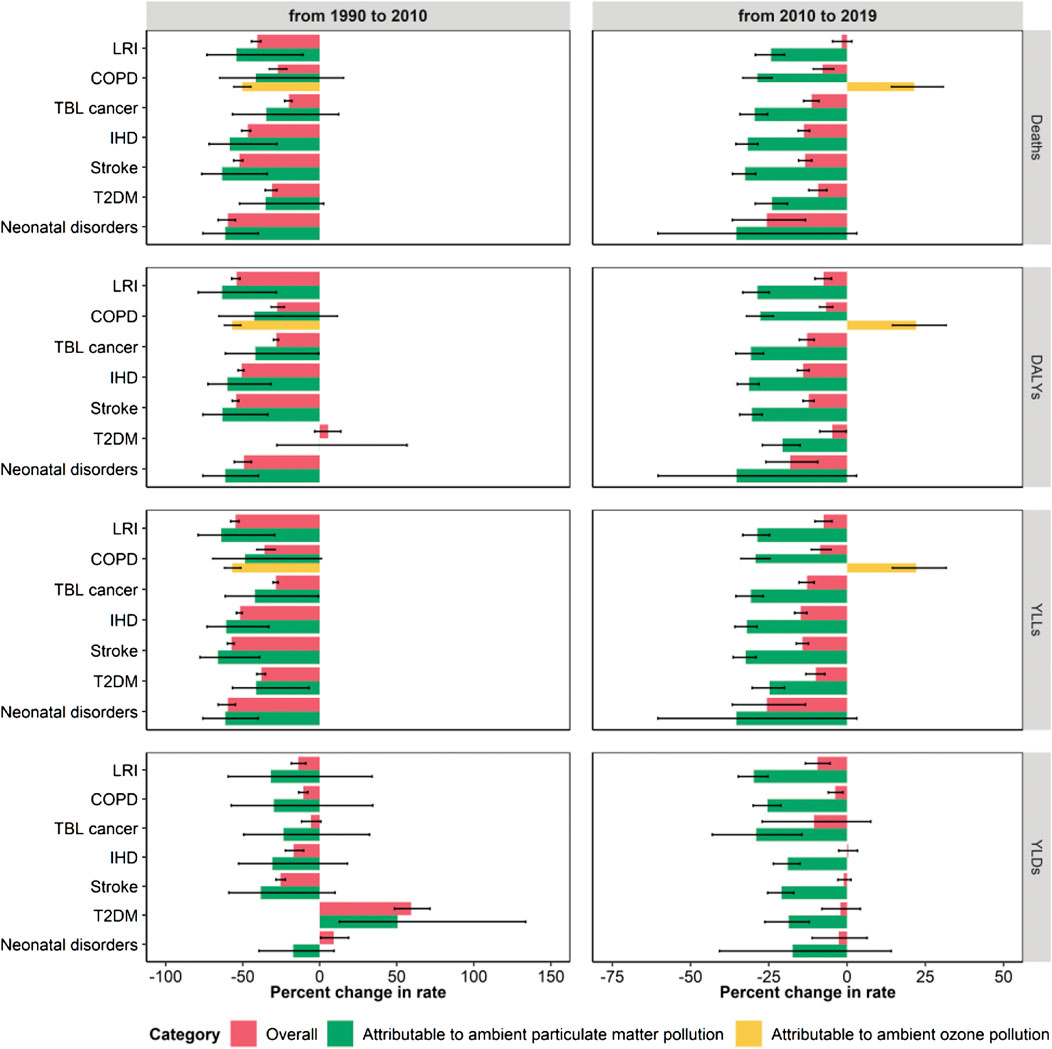
Figure 5. Estimated percent change in age-standardized rates (per 100,000 inhabitants) of mortality (deaths), disability adjusted life years (DALYs), years of life lost (YLLs), and years lived in disability (YLDs) for each cause associated with ambient air pollution. Comparison among overall variations and variations in rates due to ambient particulate matter and ozone pollution, from 1990 to 2010 and from 2010 to 2019. Whiskers represent 95% Uncertainty Intervals (Global Burden of Disease Study, Italy, 1990–2019). LRI, Lower respiratory infections; COPD, Chronic obstructive pulmonary disease; TBL, Tracheal, bronchus and lung; IHD, Ischemic heart disease; T2DM, Type 2 diabetes mellitus.
The temporal dynamic changed during the following period (2010–2019): percent reductions in the overall burden measures were much lower than in the previous period for all the analyzed diseases. In some cases, age-standardized DALY rate reductions were close to 0, as for T2DM (4.7%, 95% UI: 0.2, 8.7), COPD (6.7%, 95% UI: 4.6, 8.8) and LRI (7.5%, 95% UI: 4.9, 10.3) (Figure 5; Supplementary Table S4). Similar variations were traced for YLLs and mortality rates, while for most of the diseases YLDs did not decrease significantly. Conversely, the disease burden attributable to ambient PM2.5 consistently declined and the burden attributable to ambient ozone consistently increased (Figure 5).
Discussion
PM2.5 and Ozone Attributable Burden in 2019
GBD estimates for Italy in 2019 attribute 466,530 (95% UI: 370,621, 569,934) DALYs to ambient PM2.5, which ranked eighth among risk factors of the fourth hierarchical nested level of GBD Study and yielded 24,666 (95% UI: 19,177, 30,047) deaths [24]. Ozone accounted for 39,582 (95% UI: 18,282, 61,469) DALYs, with 3,487 (95% UI: 1,597, 5,385) associated deaths [24].
For both pollutants, and all burden measures (mortality, DALYs, YLLs and YLDs), attributable crude and age-adjusted rates were higher among males than in females, and age-specific rates were higher among children under 14 and in the elderly. The burden of ambient PM2.5, in terms of age-standardized DALY rate was unevenly stratified among exposure-related diseases: IHD and T2DM absorbed 50% of the overall burden. The gap detected between males and females is essentially explained by differences in mortality rate for these diseases [23].
The temporal variation in the burden of respiratory diseases (LRI and COPD), TBL cancer, and T2DM is due to the age-associated trends in incidence and mortality, which increase with age [25–27]. For IHD and stroke, the age-related increase comes both from age-related variations in incidence and mortality, and from the use of different exposure-response functions for each 5-year age class. This accounts for higher susceptibility to effects of air pollutants among the elderly [28, 29].
As for ozone, that is so far associated with long-term COPD mortality only, considerations mirror those related to PM2.5 and COPD.
Temporal Variation in the Burden of Ambient PM2.5
In the period 1990–2019, we identified a clear decreasing trend in PM2.5-attributable burden with an overall reduction of 47.9% (95% UI: 10.3, 65.4) of the crude DALY rate. Decreases were confirmed for mortality and YLLs, but not for YLDs. Reductions in age-standardized rates were significantly higher, accounting for 65.1% (95% UI: 41.9, 76.4) in DALY rates. In the period-stratified analysis—i.e., 1990–2010 vs. 2010–2019—a significant difference between crude and age-standardized rates reductions persisted only during the second period, probably owed to the higher precision in estimates, which have narrower uncertainty intervals. These variations parallel reductions in PM2.5 concentrations observed after the introduction of key policy interventions, starting from 1988 [30–34]. The 2008 EU directive set a target value for PM2.5 of 25 μg/m3 for long-term concentrations, which was subsequently lowered to 20 μg/m3 from 1st January 2020 [31–33]. Thereafter, PM2.5 concentrations continued to decrease with a percentage reduction of about 37% between 1990 and 2018 [35].
The observed reduction in PM2.5 concentrations leads the decreasing trend detected in the related disease burden. However, reduction of exposure is only one of the drivers of the observed trend: temporal changes are also affected by demographic and epidemiologic dynamics of the population [8, 10].
The role of demographic dynamic was analyzed comparing variations of crude and age-standardized rates. The decrease observed for age-standardized rates was significantly higher than that for crude ones, meaning that the increasing proportion of elderly population counteracts the effects of exposure reduction. Worth noting is that the large difference between crude and age-standardized rates is partially explained by the different age structure of the Italian population as compared to the global one used for the standardization: in line with our observations regarding population ageing, the Italian population has a larger elderly fraction throughout the study period (Supplementary Figure S9).
We finally considered the role of the epidemiologic dynamic, which is synthesized by temporal variations in the overall burden of diseases associated with PM2.5. From 1990 to 2010, the overall mortality, DALY and YLL rates of the diseases of interest decreased significantly, except for T2DM. YLD rates showed a moderate decline, except for neonatal disorders and T2DM. These trends are mainly driven by improvement in case management of those conditions from the 1990s [36]. For instance, the advances in care of cardiovascular diseases and the reductions in their major risk factors, some progresses in diagnosis and management of TBL cancers, as well as novel therapeutic approaches for lower respiratory diseases effectively impacted on patient outcomes and quality of life [37–41]. As regards T2DM, there was an increase of medical check-ups and laboratory tests for early detection of T2DM and systematic glycemic control in the population at risk. Likewise, the use of novel treatments impacted on the prognosis and survival of diabetic patients [42, 43].
Proportional reductions in ambient PM2.5 burden closely resembled those of each investigated disease, highlighting that the reduction of PM2.5 burden was likely unrelated to a significant decrease in the exposure to the risk factor itself. This reflects the absence of an air quality standard for PM2.5, that was enforced only in late 2010 [33]. Thereafter, the overall burden of T2DM, COPD and LRI displayed only moderate reductions, and YLDs remained constant for all diseases except for LRI and COPD. PM2.5 burden proportional decrease was significantly higher than the overall one, suggesting that the reduction in exposure concentration had a significant role in decreasing the burden attributable to the pollutant.
If we interpret our results in the framework of an accountability study that aims at evaluating the impact of air quality regulations on public health [44], our results suggest that the national regulations introduced in Italy in 2010, establishing that the average annual concentration of PM2.5 should be ≤25 μg/m3 by 2015 [33], lead to a decrease in the average PM2.5 concentration (Supplementary Figure S1), that was in turn associated with a decrease in the attributable burden of air pollution-associated diseases between 2010 and 2019. These considerations encourage the enforcement of new and more stringent regulations, in line with the recently issued WHO air quality guidelines, which recommend a yearly target value of 5 μg/m3 for PM2.5 [3]. However, a proper assessment of the future impact of such interventions should carefully account for future population dynamics and their impact on air pollution-associated outcomes [45].
Temporal Variation in the Burden of Ambient Ozone
Temporal dynamics in ozone burden differed across periods: crude and age-standardized DALY rates declined by 37.0% (95% UI: 28.9, 44.5) from 1990 to 2010, but increased by 44.8% (95% UI: 35.5, 56.3) during the following period.
This mirrors the trends in ozone concentrations, that have been increasing since 2009, despite EU Directives 2002/3/EC and 2008/50/EC set a threshold of 120 µg O3/m3 as daily maximum 8-h average not to be exceeded for more than 25 times in a year [46, 47].
When comparing the variations of crude and age-standardized DALYs and mortality rates attributable to ozone, crude rates displayed a less pronounced variation. From 1990 to 2010, the decrease in age-standardized burden was close to 50% for both DALYs and mortality, but the decrease in the crude one did not exceed 30%. The increase in age-standardized burden from 2010 to 2019 was close to 25%, but the increase in the crude one exceeded 50%. This confirms the impact of population ageing.
When comparing the variations in the overall age-standardized mortality, DALYs and YLLs of COPD, with those in ozone-attributable burden, we observed a clear indication of a beneficial impact of exposure reduction during the period 1990–2010 and an opposite detrimental effect of exposure increase in the following period. As mentioned, COPD burden was characterized by a significant reduction in both time-windows of interest. During 1990–2010, such reduction was less pronounced than the reduction in ozone-attributable burden. In 2010–2019, we observed that ozone-attributable burden consistently increased, suggesting that ozone concentrations were rising counteracting the mortality decrease.
Strengths and Limitations
GBD estimates provide a unique opportunity to assess how air pollution has changed its impact on the health of the Italian population. Nonetheless, some limitations to our study should be mentioned. First, risk-outcome pairs identified for PM2.5 and ozone exclude some diseases for which evidence, although mounting, is still not strong enough, including cardiological conditions (e.g., hypertension) and neurological diseases (e.g., dementia, intellectual disability, hypertension, asthma) [8]. Worth mentioning that a preliminary global analysis on the linkage between exposures to PM2.5 and dementia estimated that the highest dementia-related burden attributable to air pollution was found in developed countries with aging population and moderate-to-high levels of PM2.5 [48]. Ostro et al. pointed out how GBD estimates are affected by variations in exposure assessment strategies and counterfactual scenarios [49], while Burnett and Cohen described how the choice of the of relative risk functions strongly impacts on the population attributable risk estimation [50]. The magnitude of risk is assumed to depend on PM2.5 mass alone, without considering its composition [8, 51]. Furthermore, there is no distinction between sexes, and for respiratory outcomes there is no distinction among age-classes, although susceptibility to air pollution might vary by sex and age [14]. In addition, the choice of TMREL is critical, as it significantly affects the estimated burden [51]: recent results confirm that adverse health effects of PM2.5 and ozone persist at levels below the current EU PM2.5 limits [52].
Our considerations regarding the significant role of exposure reduction are based on comparisons between estimated variations and their 95% UI, therefore they retain a high level of uncertainty, that would be reduced by carrying out a decomposition analysis, actually quantifying the estimated contributions of trends in mortality and in pollutant concentration [9]. This might be a future development of our analysis. Also, our conclusions regarding the impact of future regulatory efforts should be supported by an accountability study, that allows to infer the causal relationship between interventions and the burden of air pollution [53].
Moreover, estimates of pollutants concentrations were less reliable up to 2005, due to the lack of a monitoring system for PM2.5: in Italy the first monitoring stations were introduced in 2005–2006. Finally, methods used to estimate the burden did not consider the huge variations in regional concentrations of air pollutants nor the different demographic and epidemiologic dynamics of the Italian territory.
Conclusion
In Italy, the overall decrease in the estimated burden of ambient PM2.5 and ozone between 1990 and 2019 suggests a beneficial effect of air quality regulations. However, while after 2010 these regulations continued to be followed by reductions in PM2.5 concentrations, and consequently on its attributable burden, ozone concentration is on the rise. Also, population ageing leads to an increase in susceptible population, which partially counterbalances the beneficial effects of exposure reduction. Air pollution remains a major public health concern, and new regulations are essential to mitigate its future impact. Regulatory efforts should be directed towards improving the quality of the air and protecting health, as well as to tackling climate change, which is closely interlinked with air pollution and, in particular, with ground-level ozone. Likewise, further research focusing on subnational areas and country comparisons is crucial to inform future policies and strategies to mitigate the burden.
Data Availability Statement
Availability of input data varies by source. Select data are available in a public, open-access repository. Select data are available on reasonable request. Select data may be obtained from a third party and are not publicly available. All results from the study are included in the article or uploaded as Supplementary Material or are available online.
Author Contributions
Providing data or critical feedback on data sources: ALC, GD, PF, GI, and CL. Developing methods or computational machinery: SC, PC, PF, CF, SH, FM, and JV. Providing critical feedback on methods or results: SC, CF, PF, CA, DB, EC, MC, GoC, ALC, BC, PC, GmC, GD, SD, FE, DGo, DGu, SH, GI, CLV, PL, ML, FM, LM, LGM, SM, PP, FS, RS, ET, MVe, JV, and FV. Drafting the work or revising it critically for important intellectual content: SC, CF, PF, CA, LA, IA, FB-A, AB, CB, NB, DB, GaC, MC, GoC, ALC, MSC, AC, PC, GD, BA, FE, SG, DGo, DGu, SH, II, SI, GI, CLV, PL, CL, ML, FM, LGM, PM, LM, SM, PP, UP, NP, GR, FS, SS, BS, MS, ET, BU, MVa, MVe, and FV. Managing the estimation or publications process: PF. All authors contributed to the article and approved the submitted version.
Funding
The authors declare that this study received funding from the Bill and Melinda Gates Foundation with grant number OPP1152504. The funder was not involved in the study design, collection, analysis, interpretation of data, the writing of this article or the decision to submit it for publication.
Conflict of Interest
DB reports payment or honoraria for lectures, presentations, speakers bureaus, manuscript writing or educational events from Pfizer for the European Society for Paediatric Infectious Diseases 2022 Conference, outside the submitted work. LM reports financial support for the present manuscript from the Italian Ministry of Health for the project “Ricerca Corrente 34/2017” as payments made to institution (Institute for Maternal and Child Health IRCCS Burlo Garofolo), which is a public institute. NP reports Payment or honoraria for lectures, presentations, speakers bureaus, manuscript writing or educational events, and support for attending meetings and/or travel from Bayer AG as personal payments. GR reports consulting fees from Akebia Pharmaceuticals, AstraZeneca, Alexion Pharmaceutical, BioCryst Pharmaceuticals, Silence Therapeutics, and Janssen R&D LLC; payment or honoraria for lectures, presentations, speakers bureaus, manuscript writing or educational events from Boehringer Ingelheim and Novartis as travel reimbursements; all outside the submitted work.
The remaining authors declare that they do not have any conflicts of interest.
Acknowledgments
Results of this research were presented in part at the COST Action CA18218—European Burden of Disease Network, 3rd WG Meeting, 25-26 January 2022.
Supplementary Material
The Supplementary Material for this article can be found online at: https://www.ssph-journal.org/articles/10.3389/ijph.2023.1605959/full#supplementary-material
References
1. Thurston, GD, Kipen, H, Annesi-Maesano, I, Balmes, J, Brook, RD, Cromar, K, et al. A Joint ERS/ATS Policy Statement: what Constitutes an Adverse Health Effect of Air Pollution? an Analytical Framework. Eur Respir J (2017) 49(1):1600419. doi:10.1183/13993003.00419-2016
2. World Health Organization. Expert Committee on Environmental Sanitation & World Health Organization. In: Air Pollution: Fifth Report of the Expert Committee on Environmental Sanitation. Geneva, Switzerland: World Health Organization (1958).
3. World Health Organization. WHO Global Air Quality Guidelines: Particulate Matter (PM2.5 and PM10), Ozone, Nitrogen Dioxide, Sulfur Dioxide and Carbon Monoxide. Geneva, Switzerland: World Health Organization (2021).
4. World Health Organization – Regional Office for Europe. Review of Evidence on Health Aspects of Air Pollution – REVIHAAP Project: Technical Report. Copenhagen, Denmark: WHO Europa (2013).
5. European Environment Agency. Directive 2008/50/EC of the European Parliament and of the Council of 21 May 2008 on Ambient Air Quality and Cleaner Air for Europe. Copenaghen: European Union (2008).
6. United States Environmental Protection Agency. National Ambient Air Quality Standards (2020). Available from: https://www.epa.gov/criteria-air-pollutants/naaqs-table (Accessed February 22, 2023).
7. GBD 2019 Risk Factors Collaborators. Global burden of 87 Risk Factors in 204 Countries and Territories, 1990-2019: a Systematic Analysis for the Global Burden of Disease Study 2019. Lancet (2020) 396(10258):1223–49. doi:10.1016/S0140-6736(20)30752-2
8. European Environment Agency. Air Pollution Due to Ozone: Health Impacts and Effects of Climate Change. European Environment Agency. (2023). Available from: https://www.eea.europa.eu/data-and-maps/indicators/air-pollution-by-ozone-2/assessment (last accessed on Feb 22, 2023).
9. Cohen, AJ, Brauer, M, Burnett, R, Anderson, HR, Frostad, J, Estep, K, et al. Estimates and 25-year Trends of the Global burden of Disease Attributable to Ambient Air Pollution: an Analysis of Data from the Global Burden of Diseases Study 2015. Lancet (2017) 389(10082):1907–18. doi:10.1016/S0140-6736(17)30505-6
10. Yin, P, Brauer, M, Cohen, AJ, Wang, H, Li, J, Burnett, RT, et al. The Effect of Air Pollution on Deaths, Disease burden, and Life Expectancy across China and its Provinces, 1990-2017: an Analysis for the Global Burden of Disease Study 2017. Lancet Planet Health (2020) 4(9):e386–e98. doi:10.1016/S2542-5196(20)30161-3
11. Istituto Nazionale di Statistica (ISTAT). Invecchiamento attivo e condizioni di vita degli anziani in Italia. Roma: ISTAT (2020).
12. Istituto Nazionale di Statistica (ISTAT). Rapporto annuale 2019: la situazione del Paese. Roma: ISTAT (2019).
13. EUROSTAT. Ageing Europe: Looking at the Lives of Older People Inthe EU – 2019 Edition. Luxembourg: Publications Office of the European Union (2019).
14. Yazdi, MD, Wang, Y, Di, Q, Requia, WJ, Wei, Y, Shi, L, et al. Long-term Effect of Exposure to Lower Concentrations of Air Pollution on Mortality Among US Medicare Participants and Vulnerable Subgroups: a Doubly-Robust Approach. Lancet Planet Health (2021) 5(10):e689–e697. doi:10.1016/S2542-5196(21)00204-7
15. Shaffer, RM, Sellers, SP, Baker, MG, de Buen Kalman, R, Frostad, J, Suter, MK, et al. Improving and Expanding Estimates of the Global burden of Disease Due to Environmental Health Risk Factors. Environ Health Perspect (2019) 127(10):105001. doi:10.1289/EHP5496
16. Stevens, GA, Alkema, L, Black, RE, Boerma, JT, Collins, GS, Ezzati, M, et al. Guidelines for Accurate and Transparent Health Estimates Reporting: the GATHER Statement. Lancet (2016) 388(10062):e19–e23. doi:10.1016/S0140-6736(16)30388-9
17. van Donkelaar, A, Martin, RV, Brauer, M, Hsu, NC, Kahn, RA, Levy, RC, et al. Global Estimates of Fine Particulate Matter Using a Combined Geophysical-Statistical Method with Information from Satellites, Models, and Monitors. Environ Sci Technol (2016) 50(7):3762–72. doi:10.1021/acs.est.5b05833
18. Istituto Nazionale di Statistica (ISTAT). Health for All - Italia 2020 (2023). Available from: https://www.istat.it/it/archivio/14562 (last accessed on Feb 22, 2023).
19. Zheng, P, Barber, R, Sorensen, RJD, Murray, CJL, and Aravkin, AY. Trimmed Constrained Mixed Effects Models: Formulations and Algorithms. J Comput Graphical Stat (2021) 30(3):544–56. doi:10.1080/10618600.2020.1868303
20. Global Burden of Disease Collaborative Network. Global Burden of Disease Study 2019 (GBD 2019) Particulate Matter Risk Curves. Seattle, United States of America: Institute for Health Metrics and Evaluation (2021). doi:10.6069/KHWH-2703
21. Global Burden of Disease Project. Global Health Data Exchange - GBD Results Tool (2023). Available from: http://ghdx.healthdata.org/gbd-results-tool (last accessed on Feb 22, 2023).
22. State of Global Air. State of Global Air (2020). Available from: https://www.stateofglobalair.org/data/#/air/plot ((last accessed on Mar 8, 2022).
23. GBD 2019 Diseases and Injuries Collaborators. Global burden of 369 Diseases and Injuries in 204 Countries and Territories, 1990-2019: a Systematic Analysis for the Global Burden of Disease Study 2019. Lancet (2020) 396(10258):1204–22. doi:10.1016/S0140-6736(20)30925-9
24. Global Burden of Disease Project. Global Health Data Exchange - GBD Compare (2023). Available from: https://vizhub.healthdata.org/gbd-compare/ (last accessed on Feb 22, 2023).
25. GBD 2016 Lower Respiratory Infections Collaborators. Estimates of the Global, Regional, and National Morbidity, Mortality, and Aetiologies of Lower Respiratory Infections in 195 Countries, 1990–2016: a Systematic Analysis for the Global Burden of Disease Study 2016. Lancet Infect Dis (2018) 18(11):1191–210. doi:10.1016/S1473-3099(18)30310-4
26. Global Burden of Disease Cancer Collaboration Fitzmaurice, C, Abate, D, Abbasi, N, Abbastabar, H, Abd-Allah, F, et al. Global, Regional, and National Cancer Incidence, Mortality, Years of Life Lost, Years Lived with Disability, and Disability-Adjusted Life-Years for 29 Cancer Groups, 1990 to 2017. A Systematic Analysis for the Global Burden of Disease Study. JAMA Oncol (2019) 5(12):1749–68. doi:10.1001/jamaoncol.2019.2996
27. Munshi, MN, Meneilly, GS, Rodríguez-Mañas, L, Close, KL, Conlin, PR, Cukierman-Yaffe, T, et al. Diabetes in Ageing: Pathways for Developing the Evidence Base for Clinical Guidance. Lancet Diabetes Endocrinol (2020) 8(10):855–67. doi:10.1016/S2213-8587(20)30230-8
28. Boezen, HM, Vonk, JM, van der Zee, SC, Gerritsen, J, Hoek, G, Brunekreef, B, et al. Susceptibility to Air Pollution in Elderly Males and Females. Eur Respir J (2005) 25:1018–24. doi:10.1183/09031936.05.00076104
29. Aga, E, Samoli, E, Touloumi, G, Anderson, HR, Cadum, E, Forsberg, B, et al. Short-term Effects of Ambient Particles on Mortality in the Elderly: Results from 28 Cities in the APHEA2 Project. Eur Respir J Suppl (2003) 40:28s–33s. doi:10.1183/09031936.03.00402803
30. European Environment Agency. Urban PM2.5 Concentrations Presented as Multi-Annual Average in the EU, 2008–2010. EEA (2012). Available from: https://www.eea.europa.eu/data-and-maps/figures/urban-pm2.5-concentrations-presented-as (last accessed on Feb 22, 2022).
31. Organisation for Economic Co-operation and Development (OECD). Air Pollution Exposure. OECD: OECD data (2020). Available from: https://data.oecd.org/air/air-pollution-exposure.htm ((last accessed on Feb 22, 2023).
32. Istituto superiore per la protezione e la ricerca ambientale (ISPRA). Analisi dei trend dei principali inquinanti atmosferici in Italia (2008 – 2017). Roma: ISPRA (2018). Available from: https://www.isprambiente.gov.it/files2019/pubblicazioni/rapporti/R_302_18_TREND_ARIA.pdf ((last accessed on Feb 22, 2023).
33. Gazzetta Ufficiale. Decreto Legislativo 13 agosto 2010, n.155 “Attuazione della direttiva 2008/50/CE relativa alla qualità dell'aria ambiente e per un'aria più pulita in Europa” (2010). Gazzetta Ufficiale della Repubblica Italiana n. 216 del 15. Rome: stituto Poligrafico e Zecca dello Stato.
34. Viviano, G, and Settimo, G. Normativa sulla qualità dell'aria e recepimento delle direttive della Unione Europea. Ann Ist Super Sanità (2003) 39(3):343–50.
35. Istituto superiore per la protezione e la ricerca ambientale (ISPRA). Italian Emission Inventory 1990-2018. Informative Inventory Report 2020. Roma: ISPRA (2020). Available from: https://www.isprambiente.gov.it/it/pubblicazioni/rapporti/inventario-nazionale-delle-emissioni-in-atmosfera-1990-2018.-informative-inventory-report-2020 ((last accessed on Feb 22, 2022).
36. GBD 2017 Italy Collaborators. Italy's Health Performance, 1990-2017: Findings from the Global Burden of Disease Study 2017. Lancet Public Health (2019) 4(12):e645–e657. doi:10.1016/S2468-2667(19)30189-6
37. Cortesi, PA, Fornari, C, Madotto, F, Conti, S, Naghavi, M, Bikbov, B, et al. Trends in Cardiovascular Diseases burden and Vascular Risk Factors in Italy: The Global Burden of Disease Study 1990-2017. Eur J Prev Cardiol (2020) 28:385–96. doi:10.1177/2047487320949414
38. Palmieri, L, Bennett, K, Giampaoli, S, and Capewell, S. Explaining the Decrease in Coronary Heart Disease Mortality in Italy between 1980 and 2000. Am J Public Health (2010) 100(4):684–92. doi:10.2105/AJPH.2008.147173
39. Santalucia, P, Baviera, M, Cortesi, L, Tettamanti, M, Marzona, I, Nobili, A, et al. Epidemiologic Trends in Hospitalized Ischemic Stroke from 2002 to 2010: Results from a Large Italian Population-Based Study. J Stroke Cerebrovasc Dis (2015) 24(8):1917–23. doi:10.1016/j.jstrokecerebrovasdis.2015.05.008
40. Associazione Italiana di Oncologia Medica. I Numeri del Cancro in Italia 2020. Milano: AIOM (2020). Available from: https://www.aiom.it/wp-content/uploads/2020/10/2020_Numeri_Cancro-operatori_web.pdf ((last accessed on Feb 22, 2023).
41. Pesce, G. Mortality Rates for Chronic Lower Respiratory Diseases in Italy from 1979 to 2010: an Age–Period–Cohort Analysis. ERJ Open Res (2016) 2:00093–2015. doi:10.1183/23120541.00093-2015
42. Istituto nazionale di statistica (ISTAT). Il Diabete in Italia – Anni 2000-2011. Rome: ISTAT (2012). Available from: https://www.istat.it/it/files//2012/09/Il-diabete-in-Italia.pdf ((last accessed on Feb 22, 2023).
43. Istituto nazionale di statistica (ISTAT). Il Diabete in Italia – Anni 2000-2016. Rome: ISTAT (2017). Available from: https://www.istat.it/it/files/2017/07/REPORT_DIABETE.pdf ((last accessed on Feb 22, 2023).
44. Boogaard, H, van Erp, AM, Walker, KD, and Shaikh, R. Accountability Studies on Air Pollution and Health: the HEI Experience. Curr Environ Health Rep (2017) 4(4):514–22. doi:10.1007/s40572-017-0161-0
45. GBD MAPS Working Group. Burden of Disease Attributable to Coal-Burning and Other Major Sources of Air Pollution in China. Special Report 20. Boston, MA: Health Effects Institute (2016).
46. European Environment Agency. Air Quality in Europe - 2020 Report. Luxembourg: Publications Office of the European Union (2020).
47. Gazzetta Ufficiale. Decreto Legislativo 21 Maggio 2004, n. 183 “Attuazione Della Direttiva 2002/3/CE Relativa All'ozono Nell'aria” (2004). Gazzetta Ufficiale della Repubblica Italiana n. 171 del 23. Rome: stituto Poligrafico e Zecca dello Stato.
48. Ru, M, Brauer, M, Lamarque, JF, and Shindell, D. Exploration of the Global Burden of Dementia Attributable to PM2.5: What Do We Know Based on Current Evidence? Geohealth (2021) 5(5):e2020GH000356. doi:10.1029/2020GH000356
49. Ostro, B, Spadaro, JV, Gumy, S, Mudu, P, Awe, Y, Forastiere, F, et al. Assessing the Recent Estimates of the Global burden of Disease for Ambient Air Pollution: Methodological Changes and Implications for Low- and Middle-Income Countries. Environ Res (2018) 166:713–25. doi:10.1016/j.envres.2018.03.001
50. Burnett, R, and Cohen, A. Relative Risk Functions for Estimating Excess Mortality Attributable to Outdoor PM2.5 Air Pollution: Evolution and State-Of-The-Art. Atmosphere (2020) 11(6):589. doi:10.3390/atmos11060589
51. Boogaard, H, Walker, K, and Cohen, AJ. Air Pollution: the Emergence of a Major Global Health Risk Factor. Int Health (2019) 11(6):417–21. doi:10.1093/inthealth/ihz078
52. Di, Q, Wang, Y, Zanobetti, A, Koutrakis, P, Choirat, C, Dominici, F, et al. Air Pollution and Mortality in the Medicare Population. N Engl J Med (2017) 376(26):2513–22. doi:10.1056/NEJMoa1702747
Keywords: air pollution, particulate matter, ozone, global burden of disease, air quality regulations
Citation: Conti S, Fornari C, Ferrara P, Antonazzo IC, Madotto F, Traini E, Levi M, Cernigliaro A, Armocida B, Bragazzi NL, Cadum E, Carugno M, Crotti G, Deandrea S, Cortesi PA, Guido D, Iavicoli I, Iavicoli S, La Vecchia C, Lauriola P, Michelozzi P, Scondotto S, Stafoggia M, Violante FS, Abbafati C, Albano L, Barone-Adesi F, Biondi A, Bosetti C, Buonsenso D, Carreras G, Castelpietra G, Catapano A, Cattaruzza MS, Corso B, Damiani G, Esposito F, Gallus S, Golinelli D, Hay SI, Isola G, Ledda C, Mondello S, Pedersini P, Pensato U, Perico N, Remuzzi G, Sanmarchi F, Santoro R, Simonetti B, Unim B, Vacante M, Veroux M, Villafañe JH, Monasta L and Mantovani LG (2023) Time-Trends in Air Pollution Impact on Health in Italy, 1990–2019: An Analysis From the Global Burden of Disease Study 2019. Int J Public Health 68:1605959. doi: 10.3389/ijph.2023.1605959
Received: 06 March 2023; Accepted: 17 May 2023;
Published: 02 June 2023.
Edited by:
Hanna Boogaard, Health Effects Institute, United StatesReviewed by:
Aaron Cohen, Health Effects Institute, United StatesOtto Hänninen, National Institute for Health and Welfare, Finland
Copyright © 2023 Conti, Fornari, Ferrara, Antonazzo, Madotto, Traini, Levi, Cernigliaro, Armocida, Bragazzi, Cadum, Carugno, Crotti, Deandrea, Cortesi, Guido, Iavicoli, Iavicoli, La Vecchia, Lauriola, Michelozzi, Scondotto, Stafoggia, Violante, Abbafati, Albano, Barone-Adesi, Biondi, Bosetti, Buonsenso, Carreras, Castelpietra, Catapano, Cattaruzza, Corso, Damiani, Esposito, Gallus, Golinelli, Hay, Isola, Ledda, Mondello, Pedersini, Pensato, Perico, Remuzzi, Sanmarchi, Santoro, Simonetti, Unim, Vacante, Veroux, Villafañe, Monasta and Mantovani. This is an open-access article distributed under the terms of the Creative Commons Attribution License (CC BY). The use, distribution or reproduction in other forums is permitted, provided the original author(s) and the copyright owner(s) are credited and that the original publication in this journal is cited, in accordance with accepted academic practice. No use, distribution or reproduction is permitted which does not comply with these terms.
*Correspondence: Pietro Ferrara, p.ferrara5@campus.unimib.it
This Original Article is part of the IJPH Special Issue “Science to Foster the WHO Air Quality Guideline Values”