- 1Department of Hygiene, Epidemiology and Medical Statistics, Medical School, National and Kapodistrian University of Athens, Athens, Greece
- 2Consejo Nacional de Investigaciones Cientificas y Tecnicas (CONICET), Universidad Tecnologica Nacional, Facultad Regional San Nicolas, San Nicolas, Argentina
- 3World Health Organization (WHO) Regional Office for Europe, European Centre for Environment and Health, Bonn, Germany
Objectives: We performed a systematic review and meta-analysis on long-term exposure to nitrogen dioxide (NO2) and ozone (O3) with mortality, to expand evidence that informed 2021 the WHO Air Quality Guidelines and guide the Health Risks of Air Pollution in Europe project.
Methods: We included cohorts investigating NO2 and O3 mortality from all-causes, respiratory diseases, chronic obstructive pulmonary disease (COPD), acute lower respiratory infections (ALRI); and NO2 mortality from circulatory, ischemic heart, cerebrovascular diseases and lung cancer. We pooled estimates by random-effects models and investigated heterogeneity. We assessed the certainty of the evidence using the Grading of Recommendations Assessment Development approach and Evaluation (GRADE).
Results: We selected 83 studies for NO2 and 26 for O3 for the meta-analysis. NO2 was associated with all outcomes, except for cerebrovascular mortality. O3 was associated with respiratory mortality following annual exposure. There was high heterogeneity, partly explained by region and pollutant levels. Certainty was high for NO2 with COPD and ALRI, and annual O3 with respiratory mortality.
Conclusion: An increasing body of evidence, with new results from countrywide areas and the Western Pacific, supports certainty, including new outcomes.
Introduction
In 2012-2013, the WHO Regional Office for Europe, through its European Centre for Environment and Health (ECEH), coordinated the Health Risks of Air Pollution in Europe (HRAPIE) project to provide advice on suitable concentration-response functions (CRFs) and associated information for health risk assessment. Most recently, WHO ECEH coordinated the update of the WHO global air quality guidelines (AQGs) [1]. Two systematic reviews and meta-analysis on particles [2] and gases [3] health effects were part of the evidence that guided the AQG revision. With new evidence on the health effects of air pollution rapidly accumulating, an update of the HRAPIE project was prioritized by the Joint WHO/Convention Task Force on the Health Aspects of Air Pollution under the United Nations Economic Commission for Europe Convention on Long-range Transboundary Air Pollution.
Under this framework, two systematic reviews and meta-analyses were conducted for updating and expanding the evidence used in the original WHO AQG reviews [2, 3], assessing the health effects of long-term exposure to ambient particles (PM) and gases [nitrogen dioxide (NO2) and ozone (O3)] and for which the search timeline extended up to September/October 2018.
We present the results on the association between long-term exposure to gases (NO2 and O3) with mortality, expanded to include additional mortality outcomes not previously assessed [3] and specifically: circulatory, cerebrovascular, ischemic heart diseases and lung cancer mortality for NO2. The purpose is to provide up-to-date scientific evidence for the associations under investigation, including an assessment of the certainty of the evidence, and to provide quantitatively pooled effect estimates for each pollutant-outcome combination from meta-analysis based on single exposure results to help inform the selection of concentration-response functions and associated information for relevant health risk assessment.
Methods
We included general population cohorts that reported on long-term (months to years) exposure to: NO2 and all-cause mortality, mortality due to circulatory diseases, ischemic heart diseases (IHD), respiratory diseases (chronic obstructive pulmonary disease (COPD), acute lower respiratory infections (ALRI) and lung cancer; and O3 (annual or peak/warm season) and all-cause mortality, mortality due to respiratory diseases, COPD and ALRI (Supplementary Appendix S1).
We searched the PubMed and EMBASE databases for studies published from September 2018 — the last search date that informed the previous WHO AQG review, up to May 2023 (Supplementary Material S3; Appendix S2 Table A1). As the current review includes mortality due to circulatory, cerebrovascular and ischemic heart diseases for NO2 not considered previously [3], we re-assessed articles published before September 2018 that were identified but excluded in the previous systematic review [3] as they were not part of the targeted outcomes at the time and we evaluated these studies following our current protocol. An updated search was performed in January 2024 to identify articles published after May 2023 to enrich the discussion of the current report. When multiple publications using the same cohorts were identified, we selected the study reporting on the largest cohort sample size, or as a second criterion the most recent paper. A publication was not included in the meta-analysis if it reported on a specific cohort that was part of greater collaborative project and publication reporting pooled effects between multiple cohorts. We selected the publication that reported the pooled results (and extracted the pooled effect estimate) of the analyzed cohorts and not cohort specific results or cohort specific papers. This decision 1) reduces heterogeneity due to exposure assessment and statistical methods between cohorts and 2) avoids including the same (potentially large administrative) cohort many times in the meta-analysis. Only if the cohort baseline sample size differed by more than 25% or the number of additional years reported in a cohort-specific paper was equal to or greater than the number of overlapping years with those contributing to the pooled analysis, the decision was to include both papers (the pooled analysis and the cohort-specific).
We retrieved information on: publication details; study characteristics; study population; exposure details outcome assessment; exposure unit of measurement; risk estimate as measure of association with 95% confidence interval (CI) with corresponding exposure increment (Supplementary Material S1).
Effect estimates (Hazard Ratios (HR) or Relative Risks (RR) together with 95% CIs) from single pollutant models were extracted from the selected studies and were used to calculate the pooled effect estimates. When multiple estimates were reported, we selected those reported by study authors as the “main” model in the methods section in accordance with the previous reviews [2, 3]. In cases where the effect estimates were presented per increase in parts per billion (ppb), we converted them to μg/m3 using standard factors (1.88 for NO2 and 1.96 for O3) [4]. All effect estimates are reported per 10 μg/m3.
We applied a random-effects meta-analysis using the restricted maximum likelihood method for the estimation of between studies variability [3]. Heterogeneity between studies was assessed by calculating 80% prediction intervals (80% PI), the Cochran’s Q test and the I2 statistic. Publication bias was assessed by funnel plots, and the Eggers’ test if 10 studies or more contributed to the meta-analysis.
We performed subgroup analysis to investigate heterogeneity by a) WHO regions (European Region; Region of the Americas; Western Pacific Region) and b) Risk of bias assessment per domain in cases the meta-analyses included studies at high risk of bias (High vs. Low/Moderate). We conducted a meta-regression to assess the impact of the mean level of pollutant concentrations on the effect estimates, in case 10 studies or more contributed to the meta-analysis. In case of less than 10 studies, we performed a subgroup analysis to provide pooled effect estimates separately for studies with mean levels above or below the median of the reported levels’ distribution.
All analyses were performed using the “meta” package (version 6.2-0) in the R statistical software, version 4.3.2 (https://www.r-project.org/).
Individual studies, included in the meta-analysis, were evaluated for the risk of bias (RoB), using the tool developed by WHO for the AQG systematic reviews [3, 5]. For each pollutant – outcome pair, we assessed the certainty of evidence using the modified GRADE (Grading of Recommendations, Assessment, Development and Evaluation) [6], adapted by a working group of experts under the supervision of the WHO Secretariat in the context of the update of the AQGs (Supplementary Material S3; Appendix S1).
Results
Characteristics of Studies
From the search between September 2018 and May 2023, we selected 125 studies, of which 47 reported on long-term exposure to NO2 with the outcomes of interest and 17 on O3 (annual or peak/warm period) (Figure 1; Supplementary Tables S1, S2). We re-assessed the 69 articles included in the previous meta-analysis (n = 41 for NO2 and n = 20 for O3) and 23 studies on NO2 that assessed other cardiovascular outcomes or lung cancer that were not considered at the time as relevant. Through this step, we further included 36 studies for NO2 and 9 studies for O3. In total 90 studies, 83 studies for NO2 and 26 for O3 were included in the current systematic review.
Eighty-three studies reported risk estimates on NO2 and mortality outcomes [7–89] (Supplementary Material S3 Table S1). Forty studies were conducted in the European Region, 27 in the Region of the Americas and 16 studies in the Western Pacific. The sample sizes of the selected studies varied from 2,788 to 68,721,015, while several cohorts were included in multiple papers reporting for different follow up periods and sample sizes.
Twenty-six studies assessed the association between exposure to O3 and mortality outcomes (Supplementary Material S3 Table S2) [7, 9, 12, 15, 20, 41, 42, 51, 52, 54, 55, 58, 63, 64, 66–68, 72, 73, 77, 90–95]. Ten studies were from the European Region, 13 were from the Region of the Americas and three studies from the Western Pacific Region. The sample sizes of the selected studies varied from 5,652 to 68,721,015.
Detailed RoB assessment for the studies in the meta-analysis is presented in the Supplementary Material S2. For the studies retrieved from Huangfu & Atkinson [3] we adopted the previous evaluation. For the confounding domain, most of the new studies were characterized as low/moderate RoB except for nine [14, 22, 29, 35, 56, 57, 77, 87, 95] that were rated as high; for the exposure assessment domain, two studies were evaluated as high RoB [37, 83]. For the remaining domains, all selected studies were evaluated as low or moderate RoB.
Meta-Analysis Results
Nitrogen Dioxide
Fifty-four studies presented results for NO2 and all-cause mortality (Figure 2; Table 1), out of which 34 were included in the meta-analysis, indicating that a 10 μg/m3 increase was associated with a RR of 1.05 (95% CI: 1.03, 1.07). There was high heterogeneity (80% PI: 0.98, 1.12 and I2 = 95%) that contributed to statistically significant funnel plot asymmetry (Supplementary Material S3 Figure S1). RRs were consistently >1 for almost all studies (32 out of 34). Meta-analysis by WHO region (Supplementary Material S3 Figure S2) estimated an RR of 1.03 for the European Region (n = 13), 1.03 for the Region of the Americas (n = 11), while a higher effect estimate was detected for the Western Pacific Region (RR = 1.10, n = 10). Stratification by RoB for confounding resulted in a RR 1.05 for 30 low/moderate RoB vs. 1.02 (Supplementary Material S3 Figure S3) in high RoB studies. Additional subgroup analysis, to further explore heterogeneity in all-cause mortality results, was done according to whether the cohorts were based on administrative databases (n = 7) or collected detailed individual data (n = 27), that indicated statistically significantly higher effects for the detailed cohorts (1.06 vs. 1.02, Supplementary Material S3 Figure S4). Meta-regression on NO2 levels did not explain the observed heterogeneity (p-value for levels = 0.103).
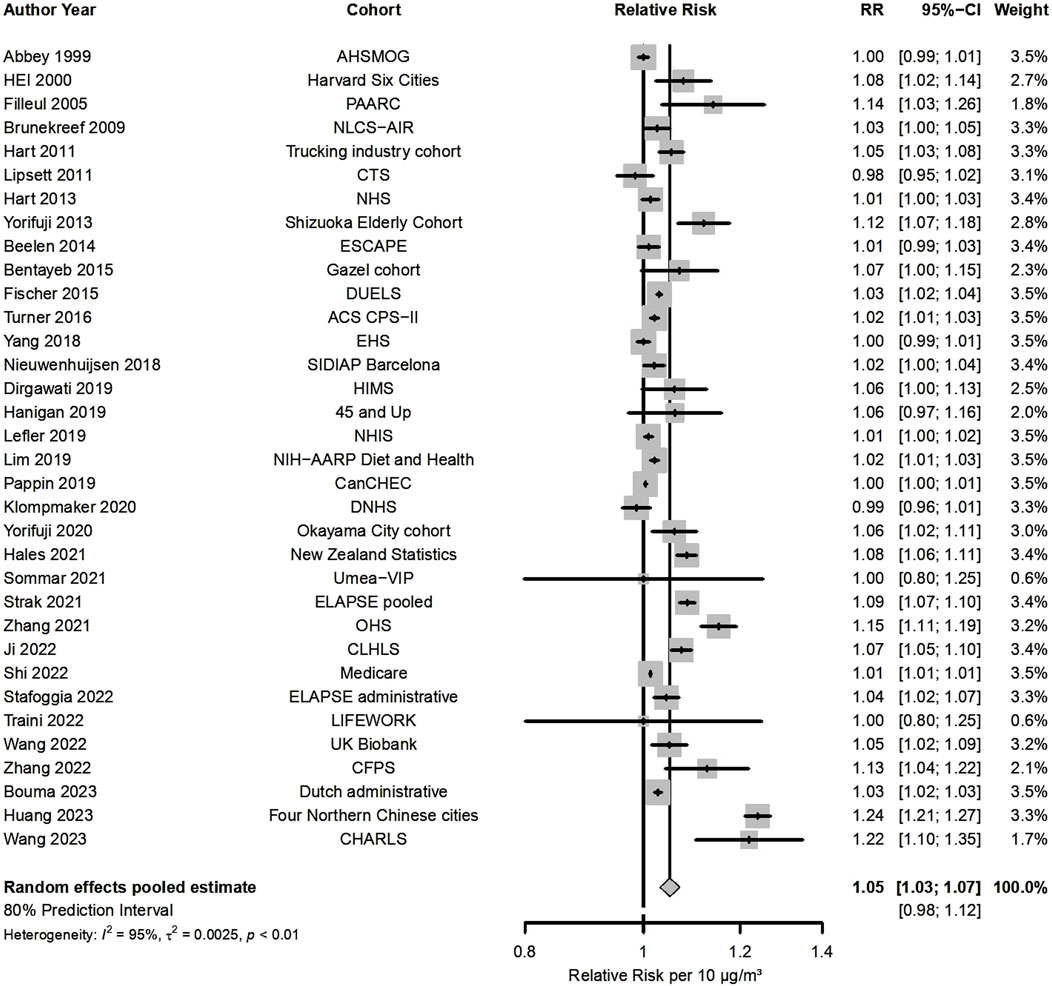
Figure 2. Forest plot for the associations between 10 μg/m3 increase in long-term exposure to nitrogen dioxide (NO2) and all-cause mortality (Global, 2023-2024).
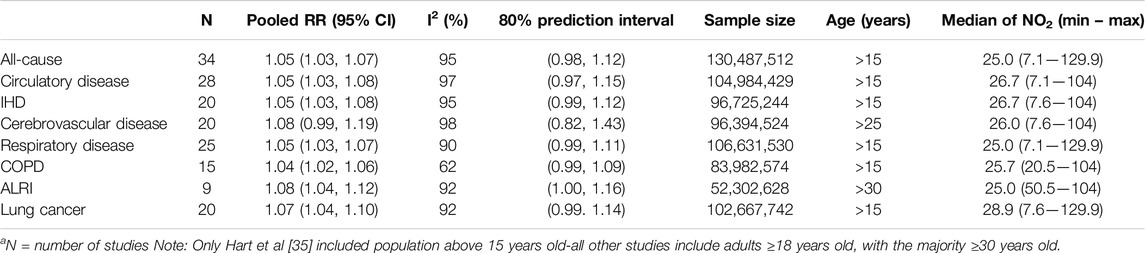
Table 1. Meta-analysis results and characteristics of studies informing the associations between long-term exposure to nitrogen dioxide (NO2) (per 10 μg/m3 increase) and mortality outcomes (Global, 2023-2024).
Twenty-eight studies estimated a RR 1.05 (95% CI: 1.03, 1.08) (Table 1; Supplementary Material S3 Figure S5). Very high heterogeneity was present (80% PI: 0.97, 1.15 and I2 = 97%) with no indication of funnel plot asymmetry (Supplementary Material S3 Figure S6). For the 12 studies in the European Region the RR was 1.02, for the region of the Americas (n = 9) and for the Western Pacific Region (n = 7) the correspondent RRs were 1.06 and 1.10, respectively (Supplementary Material S3 Figure S7). The 26 studies characterized as low/moderate RoB for confounding estimated a higher effect estimate (RR = 1.06) vs. the two high RoB studies (RR = 1.02) (Supplementary Material S3 Figure S8). Heterogeneity was not explained by NO2 levels (meta-regression p-value = 0.329).
Thirty-one studies reported results regarding exposure to NO2 and IHD mortality from which 20 were included in the meta-analysis estimating, a pooled RR 1.05 (95% CI: 1.03, 1.08) (Table 1). Heterogeneity was very high (Supplementary Material S3 Figure S9). No evidence of small study bias/funnel plot asymmetry was detected (Supplementary Material S3 Figure S10). Stratification by WHO region resulted to a RR of 1.02 for the seven studies that conducted in the European Region, 1.06 for the 9 studies from the Region of the Americas and 1.10 for the four studies from the Western Pacific Region (Supplementary Material S3 Figure S11). All studies were characterized as low/moderate risk of bias, while NO2 levels did not explain heterogeneity (meta-regression p-value = 0.467).
From the 31 studies that reported on NO2 and cerebrovascular mortality, 20 studies were included in the meta-analysis indicating that a RR of 1.08 (95% CI: 0.99, 1.19) with very high heterogeneity (Table 1; Supplementary Material S3 Figure S12). No evidence of small study bias/funnel plot asymmetry was found (Supplementary Material S3 Figure S13). Meta-analysis stratified by WHO region reported a RR of 1.04 for the European Region (n = 7), 1.01 for the Region of the Americas (n = 7), and 1.23 for the Western Pacific Region (n = 6) (Supplementary Material S3 Figure S14). One study was characterized as high risk of bias for confounding with RR 2.44 (vs. 1.04 for the rest (Supplementary Material S3 Figure S15). NO2 levels did not explain heterogeneity (meta-regression p-value = 0.870)
Thirty-five studies reported on NO2 and respiratory mortality and included in the meta-analysis estimating a RR 1.05 (95% CI: 1.03, 1.07) (Table 1; Supplementary Material S3 Figure S16) with very high heterogeneity. No evidence of small study bias/funnel plot asymmetry was found (Supplementary Material S3 Figure S17). The RR for the European Region (n = 8) was of 1.05, for the Region of the Americas 1.04 (n = 10) and 1.08 for the Western Pacific Region (n = 7) (Supplementary Material S3 Figure S18). The RR in the 23 low/moderate RoB studies was 1.05 vs. a 1.06 in high risk (n = 2) (Supplementary Material S3 Figure S19). Meta-regression indicated that 33.55% of the heterogeneity was explained by NO2 levels (p-value = 0.012) with decreasing effects for increasing levels.
Twenty studies reported effect estimates for NO2 and COPD, from which 15 were included in the meta-analysis, estimating RR 1.04 (95% CI: 1.02, 1.06) with substantial heterogeneity (Table 1; Supplementary Material S3 Figure S20). No evidence of small study bias/funnel plot asymmetry was detected (Supplementary Material S3 Figure S21). Stratification by WHO region (Supplementary Material S3 Figure S22) estimated RR 1.06 for the European Region (n = 4), 1.04, for the Region of the Americas (n = 7) and 1.01 for the Western Pacific Region (n = 4). Twelve studies evaluated as low/moderate RoB for confounding had RR 1.03 vs. 1.07 in high RoB ones (Supplementary Material S3 Figure S23). Excluding the high risk of bias studies significantly reduced heterogeneity and the PI did not include unity. NO2 levels did not explain heterogeneity (meta-regression p-value = 0.773).
Nine out of 11 studies assessing NO2 and ALRI mortality were included in the meta-analysis estimating RR 1.08 (95% CI: 1.04, 1.12) (Table 1; Supplementary Material S3 Figure S24) with very high heterogeneity, but no indication of publication bias (Supplementary Material S3 Figure S25). Only one study was conducted in the European Region (RR = 1.10). The four studies from the Region of the Americas had RR 1.10, and four studies from the Western Pacific Region 1.05 (Supplementary Material S3 Figure S26). All included studies were characterized as low/moderate for RoB. Studies with mean levels of NO2 lower than 21 μg/m3 (n = 4) estimated RR 1.10 versus 1.04 for those with levels equal or above 21 μg/m3 (n = 5) (Supplementary Material S3 Figure S27).
Twenty out of 31 studies identified for the association between NO2 and lung cancer mortality were included in the meta-analysis, and estimated RR 1.07 (95% CI: 1.04, 1.10) with high heterogeneity (Table 1; Supplementary Material S3 Figure S28) but no indication of small study bias (Supplementary Material S3 Figure S29). The RR for the European Region (n = 8) was 1.09, 1.02 for the Region of the Americas (n = 7) and 1.13 for the Western Pacific Region (n = 5) (Supplementary Material S3 Figure S30). One study was characterized as high risk of bias for confounding (RR = 1.27), while excluding this study the pooled RR was 1.06 (Supplementary Material S3 Figure S31). Meta-regression on NO2 levels indicated that 21.44% of the heterogeneity could be explained (p-value = 0.054), with increasing effects with increasing levels.
We extracted information on the shape of the concentration-response function for each exposure-outcome pair. Most studies used splines (natural or penalized splines) with varying degrees of freedom and compared to the linear model by model fit criteria, such as the model likelihood and BIC. All studies indicated increasing risk with increasing NO2 levels for all pairs, while the vast majority indicated linear or supra-linear shapes, i.e., linear shapes with steeper slopes at low pollutant levels (Supplementary Appendix S3).
Eighteen studies adjusted for PM2.5 [11, 15, 25, 30, 33, 44, 46, 51, 53, 55, 58, 67, 68, 76, 79, 80, 84, 85]; one for PM10 [28]; nine for O3 [14, 40, 51, 55, 66–68, 76, 84]; four for black carbon/smoke [22, 30, 68, 79]; two for PM2.5-10 [11, 51] and SO2 [22, 46], and one for each of SO4 [42] and CO [51]. In most of the studies, further adjustment for other pollutants did not affect the effect estimate of interest. Only five studies reported a significant impact on the estimates of interest (Supplementary Appendix S3)
Certainty of evidence assessments for NO2 and mortality outcomes are presented in the Supplementary Tables S3–S11. Most associations were assessed as moderate certainty, while COPD and ALRI mortality were assessed as high certainty (pooled RRs 1.04 and 1.08 correspondingly) and cerebrovascular mortality as low (RR = 1.08). Specifically, for all-cause mortality, 32 out of 34 studies estimated RR above unity and although the Egger’s test indicated funnel plot asymmetry the certainty in the evidence was not downgraded, as this was attributed to heterogeneity in the magnitude of the RRs. Heterogeneity was partly explained by regional differences, the high risk of bias studies, and whether the cohorts were based on administrative databases or were cohorts with detailed individual data. For COPD mortality, although there was high heterogeneity as reflected by the large PI, certainty was not downgraded, as when excluding the high risk of bias studies the PI no longer included unity.
Ozone
Nine studies reported on annual O3 and all-cause mortality contributing to a pooled RR of 1.01 (95% CI: 0.96, 1.06) (Table 2; Figure 3; Supplementary Material S3 Figure S32). There was very high heterogeneity, while the funnel of indicated no sign of asymmetry (Supplementary Material S3 Figure S33). Meta-analysis stratified by WHO region (Supplementary Material S3 Figure S34) reported an RR of 0.92 for the European Region (n = 2), 1.00 Region of the Americas (n = 5) and a higher effect estimate was detected for the Western Pacific Region (RR = 1.10, n = 2). All studies were low/moderate risk of bias, while heterogeneity was not explained by O3 levels (meta-regression p-value = 0.501).
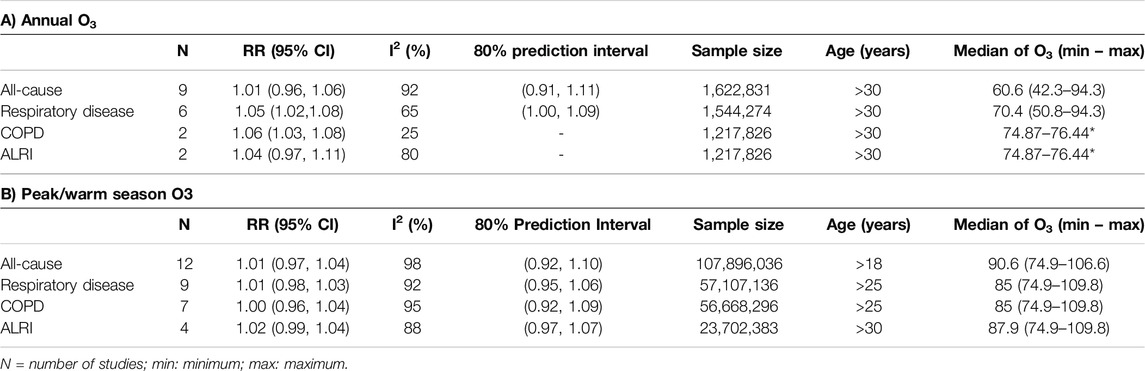
Table 2. Meta-analysis results and characteristics of studies informing the associations between long-term exposure to ozone (O3) (per 10 μg/m3 increase) and mortality outcomes (Global, 2023-2024).
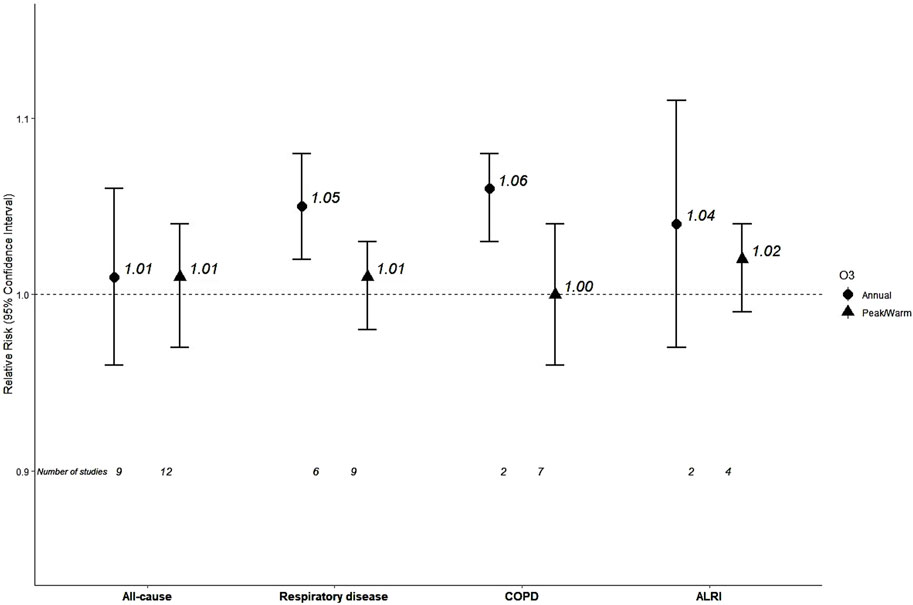
Figure 3. Pooled effect estimates for associations between 10 μg/m3 increase in long-term exposure to annual or peak/warm season ozone (O3) and mortality outcomes (Global, 2023-2024): COPD, Chronic Obstructive Pulmonary Disease; ALRI, Acute Lower Respiratory Infections.
Seven studies reported results for O3 and respiratory mortality from which six contributed in the meta-analysis that estimated RR of 1.05 (95% CI: 1.02, 1.08) for mortality from respiratory diseases (Table 2; Supplementary Material S3 Figure S35). Heterogeneity was substantial and no evidence of small study bias/funnel plot asymmetry was found (Supplementary Material S3 Figure S36). One study was in European Region (RR = 0.79), four studies in the Region of the Americas with RR 1.04 and one with RR 1.20 (95% CI: 1.02, 1.41) in the Western Pacific Region (Supplementary Material S3 Figure S37). All studies were characterized as low/moderate risk of bias. Studies with levels below 69 μg/m3 (n = 3) estimated an RR of 1.10 versus 1.04 for those equal or above 69 μg/m3 (n = 3) with no statistically significant difference (p = 0.43, Supplementary Material S3 Figure S38).
The polled estimated of two studies was 1.06 (95% CI: 1.03, 1.08) for COPD mortality, with low heterogeneity (I2 = 25%) (Table 2; Supplementary Figure S39).
The polled estimated of two studies was 1.04 (95% CI: 0.97, 1.11) for ALRI mortality, with substantial heterogeneity (I2 = 80%) (Table 2; Supplementary Material S3 Figure S40).
Twelve out of 23 studies reporting on peak/warm season O3 and all-cause mortality, estimated a pooled 1.01 (95% CI: 0.97, 1.04) for mortality from all-causes (Table 2; Supplementary Material S3 Figure S41), with very high heterogeneity. There were no signs of publication bias/funnel plot asymmetry (Supplementary Material S3 Figure S42). Meta-analysis stratified by WHO region (Supplementary Material S3 Figure S43) reported an RR of 0.93 for the European Region (n = 3), 1.01 (for the Region of the Americas (n = 6), and a higher effect estimate for the Western Pacific Region (RR = 1.07, n = 3). Stratification by RoB for confounding resulted in an RR of 1.00 for low/moderate RoB studies (n = 11) vs. one high RoB study with an RR of 1.07 (Supplementary Material S3 Figure S44). Meta-regression on 11 studies reporting O3 levels indicated that a statistically significant 25.30% of the observed heterogeneity was explained by peak/warm O3 levels (p = 0.041), with increased risk associated with increased levels.
Nine from the 15 studies that reported on peak/warm O3 and respiratory mortality, estimated an RR of 1.01 (95% CI: 0.98, 1.03) (Table 2; (Supplementary Material S3 Figure S45) with very high heterogeneity, but no evidence of funnel plot asymmetry (Supplementary Material S3 Figure S46). The meta-analysis by WHO region (Supplementary Material S3 Figure S47) estimated an RR of 0.93 for the European Region (n = 2), 1.02 for the Region of the Americas (n = 6) and 1.13 for the Western Pacific Region (n = 1). Stratification by RoB for confounding estimated an RR of 1.00 for the 8 low/moderate RoB studies vs. 1.02 for the high risk of bias study (n = 1) (Supplementary Material S3 Figure S48). Studies with mean levels of peak/warm O3 lower than 85 μg/m3 (n = 4) estimated an RR of 1.02 versus 0.99 for those with levels equal to or above 85 μg/m3 (n = 5) (p for difference = 0.240, Supplementary Material S3 Figure S49).
Seven out of eight studies that reported effect estimates for peak/warm O3 and COPD mortality estimated an RR of 1.00 (95% CI: 0.96, 1.04) with high very heterogeneity (Table 2, Supplementary Material S3 Figure S50) and no evidence of funnel plot asymmetry (Supplementary Material S3 Figure S51). The RR for the European Region (n = 2) was 0.91, while it was 1.02 for the Region of the Americas (n = 4, Supplementary Material S3 Figure S52). All selected studies were characterized as low/moderate RoB. Studies with mean levels lower than 85 μg/m3 (n = 3) estimated an RR of 1.02 versus 0.97 (n = 4) (p for difference = 0.36, Supplementary Material S3 Figure S53).
Four studies associated a 10 μg/m3 increase in peak/warm O3 with an RR of 1.02 (95% CI: 0.99, 1.04) for ALRI mortality with high heterogeneity (Table 2; Supplementary Material S3 Figure S54), and no indication of publication bias (Supplementary Material S3 Figure S55). Only one study was in the European Region (RR = 0.93) and the rest in the Region of the Americas with an RR of 1.02 (Supplementary Material S3 Figure S56). All included studies were low/moderate for the two studies with mean levels of lower than 88 μg/m3 estimated an RR of 1.04 versus 1.01 for the two with levels equal or above 88 μg/m3 (p for difference = 0.04, Supplementary Material S3 Figure S57).
Most of the studies assessing O3 effects applied splines (natural or penalized splines) and assessed linearity by model fit criteria, with the vast majority reporting no deviations from linearity (Supplementary Appendix S3). Among the studies reporting multi-pollutant models, the vast majority adjusted for particles and this adjustment did not considerably influence the estimates (Supplementary Appendix S3).
Certainty of the evidence assessments for O3 are summarized in Supplementary Tables S12–S18. For annual O3 the certainty was low for all-cause (RR = 1.01) and high for respiratory mortality (RR = 1.05), while for peak/warm O3 the certainty of evidence was low for all the mortality outcomes (all-cause RR = 1.01; respiratory RR = 1.01; COPD RR = 1.00; ALRI: RR = 1.02).
New Studies Published After Final Search
The updated search identified 438 articles published between May 2023 and January 2024. We assessed 36 following the initial screening and 15 fulfilled our eligibility criteria. Of these, seven [96–102], reported on NO2 and O3: three from the UK Biobank [97, 98, 101], out of which Wang et al. [101] reported larger RRs (1.12, 95%CI: 1.10, 1.14 for all-cause; 1.14, 95%CI: 1.10, 1.18 for circulatory) than those included from the UK Biobank in the meta-analysis (1.05, 95%CI: 1.01, 1.08 and 1.04, 95%CI: 0.97, 1.11 respectively). One study reported from CanCHEC [100] on NO2 and circulatory mortality but using a smaller sample than the included study in our analysis [20]. Results from two new European cohorts were published: 1) the RHINE III cohort [102], with 9,135 participants, reported estimates for all-cause mortality and NO2 (1.03, 95%CI: 0.84, 1.26 per 10 μg/m3) and annual O3 (0.91, 95%CI: 0.68, 1.21 per 10 μg/m3); and 2) an administrative Scottish cohort [96] reported RRs for NO2 and mortality outcomes, all of which were larger than the pooled RRs in our analysis but within the 80%PI, except for respiratory morality. Finally, we identified one paper using the China Family Panel with results for peak O3 and all-cause mortality [99] reporting an RR of 1.05 (95%CI: 1.01, 1.09).
Discussion
We updated the evidence base that informed the WHO global air quality guidelines for the associations between long-term exposure to NO2 and O3 and mortality outcomes with new studies published between 2018 and 2023, including large administrative cohorts and new reports from the Western Pacific Region. We further assessed findings on circulatory, cerebrovascular, ischemic heart diseases and lung cancer mortality for NO2 that were not considered in the previous review [3].The publication of findings from large consortia in different parts of the world and the analysis during the recent years of large administrative databases including country-wide populations highlight the need for an update of the associations. Our meta-analysis identified an increasing number of studies as compared to previous reviews and estimated for NO2 statistically significant RRs between 1.04 and 1.08 per 10 μg/m3 for all cause, circulatory, IHD, respiratory, COPD, ALRI and lung cancer mortality. The RR with cerebrovascular mortality was 1.08 but did not achieve the nominal level of significance. Using the adapted GRADE tool, we assessed the certainty in the evidence as being moderate in all cases except for COPD and ALRI mortality (rated as high) and for cerebrovascular mortality (rated as low).
Evidence increase also is associated with increased effect estimates compared with those reported in the review by Huangfu & Atkinson [3]: the RRs for all-cause, respiratory, COPD and ALRI mortality were 1.05, 1.05, 1.04 and 1.08 vs. 1.02, 1.03, 1.03 and 1.06 in the previous review. The difference between the two reviews is that the current update focused on general population cohorts and did not include evidence on patient populations. In the previous review, six patient cohorts were included in the NO2 all-cause mortality association (out of the 24 total studies), the exclusion of which did not alter the pooled relative risk (1.02) but does highlight the increase in the number of studies from 18 general population ones in the previous review to 34 in ours. The certainty in the evidence for an association did not change in these outcomes, except for COPD mortality that was increased from moderate to high. In our review we included cardiovascular and lung cancer mortality outcomes that were assessed in more than 20 studies and all associations were rated as of moderate certainty, except for cerebrovascular that was low.
A recent review on traffic related air pollution assessed NO2, although studies in the review and the meta-analysis were selected after scrutiny for the pollution’s source that resulted in reports highly specific on traffic [103]. The evidence base was less than a third of ours but the pooled RRs are similar as they reported RR 1.04 for all-cause, circulatory and lung cancer mortality, 1.05 for respiratory and IHD and 1.03 for COPD. The RR for stroke (the main contributor to cerebrovascular mortality as assessed in our review) was 1.01 that is much lower than 1.08 in the current report. Researchers in the traffic review adapted a modified GRADE tool for the certainty in the quality of the body of evidence using the Office of Health Assessment and Translation (OHAT) method as a guide [104]. All mortality outcomes were rated as high certainty in the association with NO2, except for stroke that was moderate and COPD that was as low [105]. Our assessment in the certainty for an association is more conservative, except for COPD, but we have an evidence base of 15 studies compared with 3 in the traffic review and the tool applied is different.
The evidence base has also increased for the associations with annual or peak/warm season O3 compared to Huangfu & Atkinson [3], but still the studies are few compared to NO2 related research. Although we estimated pooled RRs above unity for either annual or peak/warm O3 and all-cause, respiratory, COPD and ALRI mortality, only associations between annual O3 and respiratory or COPD mortality were statically significant. All associations were rated as low certainty in the evidence for an association except for annual O3 with respiratory mortality that was rated as high. This is an upgrade since Huangfu & Atkinson [3], which that reported 0.99 (95%CI: 0.89, 1.11) and low certainty for respiratory mortality. On the other hand, we rated the associations between peak/warm season O3 with all-cause mortality as low certainty, while they were previously rated as moderate. Although both reviews estimated the same RR of 1.01, the increasing number of studies in our review (i.e., 12 vs. 7 before) resulted in an increase in the width of the 95%CI of the pooled RR and inclusion of 1.
There was high heterogeneity in all results, except for NO2 and ALRI and annual O3 and respiratory mortality. The precise estimates from large administrative cohorts contributed to high I2 values, but not necessarily to the wide PIs that estimate the expected range of true effects in similar studies. Heterogeneity is expected considering that the studies were conducted in varying locations across the globe although most came from western Europe, the United States, and Canada. No studies were identified in the African Region, the Southeast Asian Region or Latin America and the Caribbean fitting our eligibility criteria. Analysis by WHO regions and exclusion of high risk of bias studies (in the confounding domain) reduced heterogeneity. Heterogeneity between WHO regions was mostly associated with the magnitude of the RR, giving comparable results between the European and the Americas’ Regions and higher effect estimates in the Western Pacific Region for all associations except for NO2 with COPD and ALRI mortality. For peak/warm season O3 the European Region’s results differed in direction from those in other areas, as they were below unity for all outcomes. Nevertheless, these European results were mainly based on the ELAPSE [15, 55, 67, 68] study that has attributed these findings to the small exposure range for O3 potentially driven by the European-wide exposure assessment. The levels of the pollutants explained heterogeneity in the association between NO2 and respiratory and ALRI mortality, with decreasing effects with increasing levels (partly attributed to supra-linear shapes or outliers in the meta-regression) and in the opposite direction with lung cancer mortality. Increasing peak/warm season O3 levels were associated with higher effects for all-cause but reduced effects for ALRI mortality (the latter in accordance with NO2). Finally, we do not expect that the age at recruitment to have contributed to the observed heterogeneity. With the exception of one study, all assessed studies involved adult populations (≥18 years old), and the majority focused on populations ≥30 years old.
At least half the studies in any of the exposure-outcome pairs investigated the shape of the concentration-response function. There was a consensus of no deviation from linearity, especially within the most commonly observed range of the exposure distribution for any of the associations. This implies increasing effects with increasing levels in all pairs, except for the case of peak/warm season O3 and mortality in Europe as estimated within the ELAPSE project that assessed a downward trend with increasing levels. For pairs that indicated increasing effects with increasing levels and no deviation from linearity most pointed towards steeper slopes at lower levels. This is of particular interest considering that for all pairs the median levels for NO2 levels reported in the various cohorts exceeded the annual WHO AQG level [1].
Among the strengths of the study is the long time period covering our search, the large number of studies especially for new outcomes and new regions of the world, the investigation of heterogeneity and the assessment of linearity. We closely followed the rationale of the previous reviews in assessing the new studies and the updated evidence. The increase in the related publications, including large collaborative projects that cover large areas and/or large administrative data sets, highlights the importance of updating relevant reviews as there is continuously emerging evidence for new health outcomes and an increase in the information from areas outside western Europe, the United States and Canada.
Despite the increasing evidence base, however, the investigation of heterogeneity sources may still be limited for some outcomes, as was the case for cause-specific mortality, or within regions, where the number of studies decreased. Heterogeneity may partly be attributed to varying exposure assessment methods used between cohorts including the use of different spatial coverage, grid resolution, and temporality.
A potential limitation in all systematic reviews (previous and current) is the choice of effect estimates from single exposure models. This is largely guided by the insufficient statistical information reported in multi-pollutant models, which complicates the ability to properly pool effects accounting for the variance-covariance structure in meta-analysis and reflecting the varying correlations across different areas. This raises concerns about the independence of the effects of the assessed pollutants. To address this concern, we descriptively followed the rationale of the authors that did report on the associations from multi-exposures models on the change in the magnitude of the associations of interest from single to multi pollutant models. It is of interest to note that if reviews were to account only for results from multi-pollutant models, this would decrease the evidence base as not all studies applied such models and the co-pollutants varied greatly (with most adjusting for PM2.5).
The above complexities, when considering various exposure-outcome pairs that may additionally present different degrees of heterogeneity, especially under a health risk assessment or a burden of disease framework, have been recognized in relevant endeavors undertaken by the WHO and the Global Burden of Disease study [105, 106]. For the purpose of this report heterogeneity only weakened certainty if it could not be partly attributed to the limited set of factors considered and there was diversity on the direction of extracted effect estimates.
Limitations are also related to specific items in the Risk of Bias and the adapted GRADE tool [2, 3]. Most notably the Rob tool includes the assessment of exposure contrast that is rated as low if between-subject’ variability is larger than within-subject variability. This kind of information is not reported in published papers and could not be assessed. A smaller limitation in the RoB tool is that, depending on the design of the studies and considering the growing literature using country-wide assessments, the list of proposed confounders may be limited in identifying high risk of bias studies, as it is based on known risk factors but does not account for spatial confounding that may be particularly important in air pollution research as country-wide investigations should also adjust for potential regional differences. Regarding the use of the modified GRADE, the risks in air pollution epidemiology are considerably small compared to other risk factors and may be affected disproportionally by several factors in terms of observed heterogeneity. Similar concerns were raised by the traffic review panel [103, 107]. They noted that heterogeneity in the magnitude of effect estimates should generally not weaken the certainty in the evidence, as applied in the GRADE domain on inconsistency. They proposed that rather certainty should be strengthened, if previous evidence greatly points to the same direction of effects and called for a modification of GRADE-type frameworks to align better with features of environmental health questions and related studies. Initially we have opted to account for this rationale if part of the heterogeneity could be explained by our analysis and all evidence was consistent in direction, but this was not the case in any of the examined associations.
Although all aforementioned limitations do not limit the certainty in the associations, they warrant further development. Last but not least and considering the exponentially growing literature in air pollution epidemiology, it is necessary to acknowledge that publication time does not reflect the most recent research findings, as research is bound to delays due to data availability but also necessary time for analysis and interpretation. Nevertheless, considering the support for mostly linear or supra-linear associations in our review, potentially decreasing levels of pollution in recent years do not undermine our conclusions.
Our review and meta-analysis revealed a large number of new studies investigating long-term exposure to gases and mortality, including large consortia covering greater areas, administrative datasets that in many cases represent country-wide populations and studies from the Western Pacific Region. Our results reinforce the scientific community’s call [108] for the implementation of emission reduction policies aimed at meeting the WHO AQGs. The growing evidence and the investigation of new health outcomes highlight the importance of updating reviews of air pollution epidemiology to strengthen and expand the certainty in air pollution health effects and provide updated estimates to feed into relevant health risk assessments.
Author Contributions
ES, PO, and RPV contributed to the study conception and design. RPV contributed to the project management. ES and PO developed the study protocol. PO designed the search strategy. ES, M-IK, and PO searched and assessed the studies and extracted or revised the data. ES carried out the statistical analysis and drafted the manuscript. ES, M-IK, PO, and RPV contributed to the interpretation and discussion of results. M-IK, RPV, and PO revised the manuscript and provided critical input. All authors contributed to the article and approved the submitted version.
Funding
The author(s) declare that financial support was received for the research, authorship, and/or publication of this article. This systematic review has been funded by the World Health Organization Regional Office for Europe (APW contract registration number 2023/1406752-0 and 2024/1436770-0), supported by the European Commission (DG Environment), Federal Ministry for the Environment, Nature Conservation, Nuclear Safety and Consumer Protection (Germany), Federal Office for the Environment (Switzerland) and Government of the Republic of Korea, and delivered as part of the evidence base that informs the ongoing update of the Health Risks of Air Pollution in Europe (HRAPIE-2) project.
Author Disclaimer
The authors alone are responsible for the views expressed in this article and they do not necessarily represent the views, decisions or policies of the institutions with which they are affiliated. The author affiliated with the World Health Organization (WHO) is alone responsible for the views expressed in this publication and they do not necessarily represent the decisions or policies of the WHO.
Conflict of Interest
The authors declare that they do not have any conflicts of interest.
Acknowledgments
The authors would like to recognize the valuable contributions of the WHO staff Dorota Jarosinska and Pierpaolo Mudu, HRAPIE-2 advisers Zorana Jovanovic Andersen, Olivier Chanel, Francesco Forastiere, Alison Gowers, Michal Krzyzanowski, Sylvia Medina, Martin Röösli and Joseph V. Spadaro, as well as external reviewer Richard Atkinson.
Supplementary Material
The Supplementary Material for this article can be found online at: https://www.ssph-journal.org/articles/10.3389/ijph.2024.1607676/full#supplementary-material
References
1. WHO. WHO Global Air Quality Guidelines: Particulate Matter (PM2.5 and PM10), Ozone, Nitrogen Dioxide, Sulfur Dioxide and Carbon Monoxide. Geneva: World Health Organization (2021). Available from: https://iris.who.int/handle/10665/345329 (Accessed September 12, 2024).
2. Chen, J, and Hoek, G. Long-Term Exposure to PM and All-Cause and Cause-Specific Mortality: A Systematic Review and Meta-Analysis. Environ Int (2020) 143:105974. doi:10.1016/j.envint.2020.105974
3. Huangfu, P, and Atkinson, R. Long-term Exposure to NO2 and O3 and All-Cause and Respiratory Mortality: A Systematic Review and Meta-Analysis. Environ Int (2020) 144:105998. doi:10.1016/j.envint.2020.105998
4. Defra, . UK Air: Air Information Resource. Frequently Asked Questions (2005). Available from: https://uk-air.defra.gov.uk/reports/cat06/0502160851_Conversion_Factors_Between_ppb_and.pdf (Accessed September 12, 2024).
5. WHO Regional Office for Europe. Risk of Bias Assessment Instrument for Systematic Reviews Informing WHO Global Air Quality Guidelines. Copenhagen: WHO Regional Office for Europe (2020). Available from: https://iris.who.int/handle/10665/341717 (Accessed September 12, 2024).
6. Schünemann, H, Brożek, J, Guyatt, G, and Oxman, A. GRADE Handbook for Grading Quality of Evidence and Strength of Recommendations: The GRADE Working Group (2013). Available at: http://guidelinedevelopment.org/handbook (Accessed September 12, 2024) (Updated October 2013).
7. Abbey, DE, Nishino, N, McDonnell, WF, Burchette, RJ, Knutsen, SF, Lawrence Beeson, W, et al. Long-term Inhalable Particles and Other Air Pollutants Related to Mortality in Nonsmokers. Am J Respir Crit Care Med (1999) 159(2):373–82. doi:10.1164/ajrccm.159.2.9806020
8. Andersen, ZJ, Kristiansen, LC, Andersen, KK, Olsen, TS, Hvidberg, M, Jensen, SS, et al. Stroke and Long-Term Exposure to Outdoor Air Pollution from Nitrogen Dioxide: A Cohort Study. Stroke (2012) 43(2):320–5. doi:10.1161/STROKEAHA.111.629246
9. Bauwelinck, M, Chen, J, de Hoogh, K, Katsouyanni, K, Rodopoulou, S, Samoli, E, et al. Variability in the Association between Long-Term Exposure to Ambient Air Pollution and Mortality by Exposure Assessment Method and Covariate Adjustment: A Census-Based Country-Wide Cohort Study. Sci Total Environ (2022) 804:150091. doi:10.1016/j.scitotenv.2021.150091
10. Beelen, R, Hoek, G, Houthuijs, D, van den Brandt, PA, Goldbohm, RA, Fischer, P, et al. The Joint Association of Air Pollution and Noise from Road Traffic with Cardiovascular Mortality in a Cohort Study. Occup Environ Med (2009) 66(4):243–50. doi:10.1136/oem.2008.042358
11. Beelen, R, Raaschou-Nielsen, O, Stafoggia, M, Andersen, ZJ, Weinmayr, G, Hoffmann, B, et al. Effects of Long-Term Exposure to Air Pollution on Natural-Cause Mortality: An Analysis of 22 European Cohorts within the Multicentre ESCAPE Project. Lancet (2014) 383(9919):785–95. doi:10.1016/S0140-6736(13)62158-3
12. Bentayeb, M, Wagner, V, Stempfelet, M, Zins, M, Goldberg, M, Pascal, M, et al. Association Between Long-Term Exposure to Air Pollution and Mortality in France: A 25-Year Follow-Up Study. Environ Int (2015) 85:5–14. doi:10.1016/j.envint.2015.08.006
13. Bouma, F, Janssen, NA, Wesseling, J, van Ratingen, S, Strak, M, Kerckhoffs, J, et al. Long-Term Exposure to Ultrafine Particles and Natural and Cause-Specific Mortality. Environ Int (2023) 175(no pagination):107960. doi:10.1016/j.envint.2023.107960
14. Brunekreef, B, Beelen, R, Hoek, G, Schouten, L, Bausch-Goldbohm, S, Fischer, P, et al. Effects of Long-Term Exposure to Traffic-Related Air Pollution on Respiratory and Cardiovascular Mortality in the Netherlands: The NLCS-AIR Study. Res Rep Health Eff Inst (2009)(139) 5–89. discussion 3-89. Available from: https://www.healtheffects.org/publication/effects-long-term-exposure-traffic-related-air-pollution-respiratory-and-cardiovascular
15. Brunekreef, B, Strak, M, Chen, J, Andersen, ZJ, Atkinson, R, Bauwelinck, M, et al. Mortality and Morbidity Effects of Long-Term Exposure to Low-Level PM2. 5, BC, NO2, and O3: An Analysis of European Cohorts in the ELAPSE Project. Research Reports: Health Effects Institute (2021).
16. Cesaroni, G, Badaloni, C, Gariazzo, C, Stafoggia, M, Sozzi, R, Davoli, M, et al. Long-term Exposure to Urban Air Pollution and Mortality in a Cohort of More Than a Million Adults in Rome. Environ Health Perspect (2013) 121(3):324–31. doi:10.1289/ehp.1205862
17. Chen, H, Goldberg, MS, Burnett, RT, Jerrett, M, Wheeler, AJ, and Villeneuve, PJ. Long-term Exposure to Traffic-Related Air Pollution and Cardiovascular Mortality. Epidemiology (2013) 24(1):35–43. doi:10.1097/EDE.0b013e318276c005
18. Chen, LH, Knutsen, SF, Shavlik, D, Beeson, WL, Petersen, F, Ghamsary, M, et al. The Association Between Fatal Coronary Heart Disease and Ambient Particulate Air Pollution: Are Females at Greater Risk? Environ Health Perspect (2005) 113(12):1723–9. doi:10.1289/ehp.8190
19. Chen, X, Zhang, LW, Huang, JJ, Song, FJ, Zhang, LP, Qian, ZM, et al. Long-Term Exposure to Urban Air Pollution and Lung Cancer Mortality: A 12-Year Cohort Study in Northern China. Sci Total Environ (2016) 571:855–61. doi:10.1016/j.scitotenv.2016.07.064
20. Crouse, DL, Peters, PA, Hystad, P, Brook, JR, van Donkelaar, A, Martin, RV, et al. Ambient PM2.5, O(3), and NO(2) Exposures and Associations With Mortality Over 16 Years of Follow-Up in the Canadian Census Health and Environment Cohort (CanCHEC). Environ Health Perspect (2015) 123(11):1180–6. doi:10.1289/ehp.1409276
21. Crouse, DL, Peters, PA, Villeneuve, PJ, Proux, MO, Shin, HH, Goldberg, MS, et al. Within- and Between-City Contrasts in Nitrogen Dioxide and Mortality in 10 Canadian Cities; a Subset of the Canadian Census Health and Environment Cohort (CanCHEC). J Expo Sci Environ Epidemiol (2015) 25(5):482–9. doi:10.1038/jes.2014.89
22. Dehbi, HM, Blangiardo, M, Gulliver, J, Fecht, D, de Hoogh, K, Al-Kanaani, Z, et al. Air Pollution and Cardiovascular Mortality With Over 25Years Follow-Up: A Combined Analysis of Two British Cohorts. Environ Int (2017) 99:275–81. doi:10.1016/j.envint.2016.12.004
23. Dimakopoulou, K, Samoli, E, Beelen, R, Stafoggia, M, Andersen, ZJ, Hoffmann, B, et al. Air Pollution and Nonmalignant Respiratory Mortality in 16 Cohorts Within the ESCAPE Project. Am J Respir Crit Care Med (2014) 189(6):684–96. doi:10.1164/rccm.201310-1777OC
24. Dirgawati, M, Hinwood, A, Nedkoff, L, Hankey, GJ, Yeap, BB, Flicker, L, et al. Long-term Exposure to Low Air Pollutant Concentrations and the Relationship With All-Cause Mortality and Stroke in Older Men. Epidemiology (2019) 30(Suppl. 1):S82-S89–s9. doi:10.1097/ede.0000000000001034
25. Eum, KD, Honda, TJ, Wang, B, Kazemiparkouhi, F, Manjourides, J, Pun, VC, et al. Long-Term Nitrogen Dioxide Exposure and Cause-Specific Mortality in the U.S. Medicare Population. Environ Res (2022) 207:112154. doi:10.1016/j.envres.2021.112154
26. Eum, KD, Kazemiparkouhi, F, Wang, B, Manjourides, J, Pun, V, Pavlu, V, et al. Long-Term NO(2) Exposures and Cause-Specific Mortality in American Older Adults. Environ Int (2019) 124:10–5. doi:10.1016/j.envint.2018.12.060
27. Filleul, L, Rondeau, V, Vandentorren, S, Le Moual, N, Cantagrel, A, Annesi-Maesano, I, et al. Twenty Five Year Mortality and Air Pollution: Results From the French PAARC Survey. Occup Environ Med (2005) 62(7):453–60. doi:10.1136/oem.2004.014746
28. Fischer, PH, Marra, M, Ameling, CB, Hoek, G, Beelen, R, de Hoogh, K, et al. Air Pollution and Mortality in Seven Million Adults: The Dutch Environmental Longitudinal Study (DUELS). Environ Health Perspect (2015) 123(7):697–704. doi:10.1289/ehp.1408254
29. Gan, WQ, FitzGerald, JM, Carlsten, C, Sadatsafavi, M, and Brauer, M. Associations of Ambient Air Pollution With Chronic Obstructive Pulmonary Disease Hospitalization and Mortality. Am J Respir Crit Care Med (2013) 187(7):721–7. doi:10.1164/rccm.201211-2004OC
30. Gan, WQ, Koehoorn, M, Davies, HW, Demers, PA, Tamburic, L, and Brauer, M. Long-term Exposure to Traffic-Related Air Pollution and the Risk of Coronary Heart Disease Hospitalization and Mortality. Environ Health Perspect (2011) 119(4):501–7. doi:10.1289/ehp.1002511
31. Gariazzo, C, Carlino, G, Silibello, C, Tinarelli, G, Renzi, M, Finardi, S, et al. Impact of Different Exposure Models and Spatial Resolution on the Long-Term Effects of Air Pollution. Environ Res (2021) 192:110351. doi:10.1016/j.envres.2020.110351
32. Gehring, U, Heinrich, J, Kramer, U, Grote, V, Hochadel, M, Sugiri, D, et al. Long-term Exposure to Ambient Air Pollution and Cardiopulmonary Mortality in Women. Epidemiology (2006) 17(5):545–51. doi:10.1097/01.ede.0000224541.38258.87
33. Hales, S, Atkinson, J, Metcalfe, J, Kuschel, G, and Woodward, A. Long Term Exposure to Air Pollution, Mortality and Morbidity in New Zealand: Cohort Study. Sci Total Environ (2021) 801:149660. doi:10.1016/j.scitotenv.2021.149660
34. Hanigan, IC, Rolfe, MI, Knibbs, LD, Salimi, F, Cowie, CT, Heyworth, J, et al. All-cause Mortality and Long-Term Exposure to Low Level Air Pollution in the '45 and Up Study' Cohort, Sydney, Australia, 2006-2015. Environ Int (2019) 126:762–70. doi:10.1016/j.envint.2019.02.044
35. Hart, JE, Garshick, E, Dockery, DW, Smith, TJ, Ryan, L, and Laden, F. Long-Term Ambient Multipollutant Exposures and Mortality. Am J Respir Crit Care Med (2011) 183(1):73–8. doi:10.1164/rccm.200912-1903OC
36. Hart, JE, Rimm, EB, Rexrode, KM, and Laden, F. Changes in Traffic Exposure and the Risk of Incident Myocardial Infarction and All-Cause Mortality. Epidemiology (2013) 24(5):734–42. doi:10.1097/EDE.0b013e31829d5dae
37. Heinrich, J, Thiering, E, Rzehak, P, Kramer, U, Hochadel, M, Rauchfuss, KM, et al. Long-Term Exposure to NO2 and PM10 and All-Cause and Cause-Specific Mortality in a Prospective Cohort of Women. Occup Environ Med (2013) 70(3):179–86. doi:10.1136/oemed-2012-100876
38. Heritier, H, Vienneau, D, Foraster, M, Eze, IC, Schaffner, E, de Hoogh, K, et al. A Systematic Analysis of Mutual Effects of Transportation Noise and Air Pollution Exposure on Myocardial Infarction Mortality: A Nationwide Cohort Study in Switzerland. Eur Heart J (2019) 40(7):598–603. doi:10.1093/eurheartj/ehy650
39. Hoek, G, Brunekreef, B, Goldbohm, S, Fischer, P, and van den Brandt, PA. Association between Mortality and Indicators of Traffic-Related Air Pollution in the Netherlands: A Cohort Study. Lancet (2002) 360(9341):1203–9. doi:10.1016/S0140-6736(02)11280-3
40. Huang, W, Zhou, Y, Chen, X, Zeng, X, Knibbs, LD, Zhang, Y, et al. Individual and Joint Associations of Long-Term Exposure to Air Pollutants and Cardiopulmonary Mortality: A 22-Year Cohort Study in Northern China. Lancet Reg Health West Pac (2023) 36:100776. doi:10.1016/j.lanwpc.2023.100776
41. Hvidtfeldt, UA, Sorensen, M, Geels, C, Ketzel, M, Khan, J, Tjonneland, A, et al. Long-Term Residential Exposure to PM(2.5), PM(10), Black Carbon, NO(2), and Ozone and Mortality in a Danish Cohort. Environ Int (2019) 123:265–72. doi:10.1016/j.envint.2018.12.010
42. Health Effects Institute. Reanalysis of the Harvard Six Cities Study and the American Cancer Society Study of Particulate Air Pollution and Mortality: A Special Report of the Institute’s Particle Epidemiology Reanalysis Project. Boston MA, United States: HEI (2000).
43. Jerrett, M, Burnett, RT, Beckerman, BS, Turner, MC, Krewski, D, Thurston, G, et al. Spatial Analysis of Air Pollution and Mortality in California. Am J Respir Crit Care Med (2013) 188(5):593–9. doi:10.1164/rccm.201303-0609OC
44. Ji, JS, Liu, L, Zhang, JJ, Kan, H, Zhao, B, Burkart, KG, et al. NO(2) and PM(2.5) Air Pollution Co-Exposure and Temperature Effect Modification on Pre-Mature Mortality in Advanced Age: A Longitudinal Cohort Study in China. Environ Health (2022) 21(1):97. doi:10.1186/s12940-022-00901-8
45. Katanoda, K, Sobue, T, Satoh, H, Tajima, K, Suzuki, T, Nakatsuka, H, et al. An Association Between Long-Term Exposure to Ambient Air Pollution and Mortality From Lung Cancer and Respiratory Diseases in Japan. J Epidemiol (2011) 21(2):132–43. doi:10.2188/jea.je20100098
46. Kim, H, Byun, G, Choi, Y, Kim, S, Kim, SY, and Lee, JT. Effects of Long-Term Exposure to Air Pollution on All-Cause Mortality and Cause-specific Mortality in Seven Major Cities of South Korea: Korean National Health and Nutritional Examination Surveys With Mortality Follow-Up. Environ Res (2021) 192:110290. doi:10.1016/j.envres.2020.110290
47. Klompmaker, JO, Hoek, G, Bloemsma, LD, Marra, M, Wijga, AH, van den Brink, C, et al. Surrounding Green, Air Pollution, Traffic Noise Exposure and Non-accidental and Cause-specific Mortality. Environ Int (2020) 134:105341. doi:10.1016/j.envint.2019.105341
48. Klompmaker, JO, Janssen, N, Andersen, ZJ, Atkinson, R, Bauwelinck, M, Chen, J, et al. Comparison of Associations between Mortality and Air Pollution Exposure Estimated With a Hybrid, a Land-Use Regression and a Dispersion Model. Environ Int (2021) 146:106306. doi:10.1016/j.envint.2020.106306
49. Klompmaker, JO, Janssen, NAH, Bloemsma, LD, Marra, M, Lebret, E, Gehring, U, et al. Effects of Exposure to Surrounding Green, Air Pollution and Traffic Noise With Non-Accidental and Cause-specific Mortality in the Dutch National Cohort. Environ Health (2021) 20(1):82. doi:10.1186/s12940-021-00769-0
50. Kulick, ER, Eliot, MN, Szpiro, AA, Coull, BA, Tinker, LF, Eaton, CB, et al. Long-Term Exposure to Ambient Particulate Matter and Stroke Etiology: Results From the Women's Health Initiative. Environ Res (2023) 224(no pagination):115519. doi:10.1016/j.envres.2023.115519
51. Lefler, JS, Higbee, JD, Burnett, RT, Ezzati, M, Coleman, NC, Mann, DD, et al. Air Pollution and Mortality in a Large, Representative U.S. Cohort: Multiple-Pollutant Analyses, and Spatial and Temporal Decompositions. Environ Health (2019) 18(1):101. doi:10.1186/s12940-019-0544-9
52. Lim, CC, Hayes, RB, Ahn, J, Shao, Y, Silverman, DT, Jones, RR, et al. Long-Term Exposure to Ozone and Cause-Specific Mortality Risk in the United States. Am J Respir Crit Care Med (2019) 200(8):1022–31. doi:10.1164/rccm.201806-1161OC
53. Lim, CC, Hayes, RB, Ahn, J, Shao, Y, Silverman, DT, Jones, RR, et al. Mediterranean Diet and the Association Between Air Pollution and Cardiovascular Disease Mortality Risk. Circulation (2019) 139(15):1766–75. doi:10.1161/CIRCULATIONAHA.118.035742
54. Lipsett, MJ, Ostro, BD, Reynolds, P, Goldberg, D, Hertz, A, Jerrett, M, et al. Long-term Exposure to Air Pollution and Cardiorespiratory Disease in the California Teachers Study Cohort. Am J Respir Crit Care Med (2011) 184(7):828–35. doi:10.1164/rccm.201012-2082OC
55. Liu, S, Lim, YH, Chen, J, Strak, M, Wolf, K, Weinmayr, G, et al. Long-Term Air Pollution Exposure and Pneumonia-Related Mortality in a Large Pooled European Cohort. Am J Respir Crit Care Med (2022) 205(12):1429–39. doi:10.1164/rccm.202106-1484OC
56. Naess, O, Nafstad, P, Aamodt, G, Claussen, B, and Rosland, P. Relation between Concentration of Air Pollution and Cause-Specific Mortality: Four-Year Exposures to Nitrogen Dioxide and Particulate Matter Pollutants in 470 Neighborhoods in Oslo, Norway. Am J Epidemiol (2007) 165(4):435–43. doi:10.1093/aje/kwk016
57. Nieuwenhuijsen, MJ, Gascon, M, Martinez, D, Ponjoan, A, Blanch, J, Garcia-Gil, MDM, et al. Air Pollution, Noise, Blue Space, and Green Space and Premature Mortality in Barcelona: A Mega Cohort. Int J Environ Res Public Health (2018) 15(11):2405. doi:10.3390/ijerph15112405
58. Pappin, AJ, Christidis, T, Pinault, LL, Crouse, DL, Brook, JR, Erickson, A, et al. Examining the Shape of the Association between Low Levels of Fine Particulate Matter and Mortality Across Three Cycles of the Canadian Census Health and Environment Cohort. Environ Health Perspect (2019) 127(10):107008. doi:10.1289/EHP5204
59. Qian, Y, Li, H, Rosenberg, A, Li, Q, Sarnat, J, Papatheodorou, S, et al. Long-Term Exposure to Low-Level NO2 and Mortality Among the Elderly Population in the Southeastern United States. Environ Health Perspect (2021) 129(12):127009. doi:10.1289/EHP9044
60. Raaschou-Nielsen, O, Andersen, ZJ, Jensen, SS, Ketzel, M, Sørensen, M, Hansen, J, et al. Traffic Air Pollution and Mortality From Cardiovascular Disease and All Causes: A Danish Cohort Study. Environ Health (2012) 11:60. doi:10.1186/1476-069x-11-60
61. Samoli, E, Rodopoulou, S, Hvidtfeldt, UA, Wolf, K, Stafoggia, M, Brunekreef, B, et al. Modeling Multi-Level Survival Data in Multi-Center Epidemiological Cohort Studies: Applications From the ELAPSE Project. Environ Int (2021) 147:106371. doi:10.1016/j.envint.2020.106371
62. Schikowski, T, Sugiri, D, Ranft, U, Gehring, U, Heinrich, J, Wichmann, HE, et al. Does Respiratory Health Contribute to the Effects of Long-Term Air Pollution Exposure on Cardiovascular Mortality? Respir Res (2007) 8(1):20. doi:10.1186/1465-9921-8-20
63. Shi, L, Rosenberg, A, Wang, Y, Liu, P, Danesh Yazdi, M, Requia, W, et al. Low-Concentration Air Pollution and Mortality in American Older Adults: A National Cohort Analysis (2001-2017). Environ Sci Technol (2022) 56(11):7194–202. doi:10.1021/acs.est.1c03653
64. So, R, Andersen, ZJ, Chen, J, Stafoggia, M, de Hoogh, K, Katsouyanni, K, et al. Long-Term Exposure to Air Pollution and Mortality in a Danish Nationwide Administrative Cohort Study: Beyond Mortality From Cardiopulmonary Disease and Lung Cancer. Environ Int (2022) 164:107241. doi:10.1016/j.envint.2022.107241
65. So, R, Jørgensen, JT, Lim, YH, Mehta, AJ, Amini, H, Mortensen, LH, et al. Long-Term Exposure to Low Levels of Air Pollution and Mortality Adjusting for Road Traffic Noise: A Danish Nurse Cohort Study. Environ Int (2020) 143:105983. doi:10.1016/j.envint.2020.105983
66. Sommar, JN, Hvidtfeldt, UA, Geels, C, Frohn, LM, Brandt, J, Christensen, JH, et al. Long-Term Residential Exposure to Particulate Matter and its Components, Nitrogen Dioxide and Ozone-A Northern Sweden Cohort Study on Mortality. Int J Environ Res Public Health (2021) 18(16):8476. doi:10.3390/ijerph18168476
67. Stafoggia, M, Oftedal, B, Chen, J, Rodopoulou, S, Renzi, M, Atkinson, RW, et al. Long-Term Exposure to Low Ambient Air Pollution Concentrations and Mortality Among 28 Million People: Results From Seven Large European Cohorts Within the ELAPSE Project. Lancet Planet Health (2022) 6(1):e9–e18. doi:10.1016/s2542-5196(21)00277-1
68. Strak, M, Weinmayr, G, Rodopoulou, S, Chen, J, de Hoogh, K, Andersen, ZJ, et al. Long Term Exposure to Low Level Air Pollution and Mortality in Eight European Cohorts within the ELAPSE Project: Pooled Analysis. BMJ (2021) 374:n1904. doi:10.1136/bmj.n1904
69. Tian, F, Cai, M, Li, H, Qian, ZM, Chen, L, Zou, H, et al. Air Pollution Associated With Incident Stroke, Poststroke Cardiovascular Events, and Death: A Trajectory Analysis of a Prospective Cohort. Neurology (2022) 99(22):e2474–e2484. doi:10.1212/WNL.0000000000201316
70. Traini, E, Huss, A, Portengen, L, Rookus, M, Verschuren, WMM, Vermeulen, RCH, et al. A Multipollutant Approach to Estimating Causal Effects of Air Pollution Mixtures on Overall Mortality in a Large, Prospective Cohort. Epidemiology (2022) 33(4):514–22. doi:10.1097/ede.0000000000001492
71. Tseng, E, Ho, WC, Lin, MH, Cheng, TJ, Chen, PC, and Lin, HH. Chronic Exposure to Particulate Matter and Risk of Cardiovascular Mortality: Cohort Study from Taiwan. BMC Public Health (2015) 15:936. doi:10.1186/s12889-015-2272-6
72. Turner, MC, Jerrett, M, Pope, CA, Krewski, D, Gapstur, SM, Diver, WR, et al. Long-Term Ozone Exposure and Mortality in a Large Prospective Study. Am J Respir Crit Care Med (2016) 193(10):1134–42. doi:10.1164/rccm.201508-1633OC
73. Vienneau, D, Stafoggia, M, Rodopoulou, S, Chen, J, Atkinson, RW, Bauwelinck, M, et al. Association Between Exposure to Multiple Air Pollutants, Transportation Noise and Cause-Specific Mortality in Adults in Switzerland. Environ Health (2023) 22(1):29. (no pagination. doi:10.1186/s12940-023-00983-y
74. Wang, M, Zhou, T, Song, Q, Ma, H, Hu, Y, Heianza, Y, et al. Ambient Air Pollution, Healthy Diet and Vegetable Intakes, and Mortality: A Prospective UK Biobank Study. Int J Epidemiol (2022) 51(4):1243–53. doi:10.1093/ije/dyac022
75. Wang, X, Chen, L, Cai, M, Tian, F, Zou, H, Qian, ZM, et al. Air Pollution Associated With Incidence and Progression Trajectory of Chronic Lung Diseases: A Population-Based Cohort Study. Thorax (2023) 78(7):698–705. doi:10.1136/thorax-2022-219489
76. Wang, Y, Luo, S, Wei, J, Yang, Z, Hu, K, Yao, Y, et al. Ambient NO2 Exposure Hinders Long-Term Survival of Chinese Middle-Aged and Older Adults. Sci Total Environ (2023) 855(no pagination):158784. doi:10.1016/j.scitotenv.2022.158784
77. Weichenthal, S, Pinault, LL, and Burnett, RT. Impact of Oxidant Gases on the Relationship Between Outdoor Fine Particulate Air Pollution and Nonaccidental, Cardiovascular, and Respiratory Mortality. Sci Rep (2017) 7(1):16401. doi:10.1038/s41598-017-16770-y
78. Wu, G, Cai, M, Wang, C, Zou, H, Wang, X, Hua, J, et al. Ambient Air Pollution and Incidence, Progression to Multimorbidity and Death of Hypertension, Diabetes, and Chronic Kidney Disease: A National Prospective Cohort. The Sci total Environ (2023) 881:163406. doi:10.1016/j.scitotenv.2023.163406
79. Yang, Y, Tang, R, Qiu, H, Lai, PC, Wong, P, Thach, TQ, et al. Long Term Exposure to Air Pollution and Mortality in an Elderly Cohort in Hong Kong. Environ Int (2018) 117:99–106. doi:10.1016/j.envint.2018.04.034
80. Yorifuji, T, and Kashima, S. Long-Term Exposure to Nitrogen Dioxide and Natural-Cause and Cause-Specific Mortality in Japan. Sci Total Environ (2020) 741:140465. doi:10.1016/j.scitotenv.2020.140465
81. Yorifuji, T, Kashima, S, Tsuda, T, Ishikawa-Takata, K, Ohta, T, Tsuruta, K, et al. Long-term Exposure to Traffic-Related Air Pollution and the Risk of Death From Hemorrhagic Stroke and Lung Cancer in Shizuoka, Japan. Sci Total Environ (2013) 443:397–402. doi:10.1016/j.scitotenv.2012.10.088
82. Yorifuji, T, Kashima, S, Tsuda, T, Takao, S, Suzuki, E, Doi, H, et al. Long-Term Exposure to Traffic-Related Air Pollution and Mortality in Shizuoka, Japan. Occup Environ Med (2010) 67(2):111–7. doi:10.1136/oem.2008.045542
83. Zhang, P, Dong, G, Sun, B, Zhang, L, Chen, X, Ma, N, et al. Long-term Exposure to Ambient Air Pollution and Mortality Due to Cardiovascular Disease and Cerebrovascular Disease in Shenyang, China. PLoS One (2011) 6(6):e20827. doi:10.1371/journal.pone.0020827
84. Zhang, Y, Li, Z, Wei, J, Zhan, Y, Liu, L, Yang, Z, et al. Long-term Exposure to Ambient NO(2) and Adult Mortality: A Nationwide Cohort Study in China. J Adv Res (2022) 41:13–22. doi:10.1016/j.jare.2022.02.007
85. Zhang, Z, Wang, J, Kwong, JC, Burnett, RT, van Donkelaar, A, Hystad, P, et al. Long-term Exposure to Air Pollution and Mortality in a Prospective Cohort: The Ontario Health Study. Environ Int (2021) 154:106570. doi:10.1016/j.envint.2021.106570
86. Zhang, Z, Weichenthal, S, Kwong, JC, Burnett, RT, Hatzopoulou, M, Jerrett, M, et al. Long-term Exposure to Iron and Copper in Fine Particulate Air Pollution and Their Combined Impact on Reactive Oxygen Species Concentration in Lung Fluid: A Population-Based Cohort Study of Cardiovascular Disease Incidence and Mortality in Toronto, Canada. Int J Epidemiol (2021) 50(2):589–601. doi:10.1093/ije/dyaa230
87. Zhang, Z, Weichenthal, S, Kwong, JC, Burnett, RT, Hatzopoulou, M, Jerrett, M, et al. A Population-Based Cohort Study of Respiratory Disease and Long-Term Exposure to Iron and Copper in Fine Particulate Air Pollution and Their Combined Impact on Reactive Oxygen Species Generation in Human Lungs. Environ Sci Technol (2021) 55(6):3807–18. doi:10.1021/acs.est.0c05931
88. Zhao, N, Pinault, L, Toyib, O, Vanos, J, Tjepkema, M, and Cakmak, S. Long-Term Ozone Exposure and Mortality From Neurological Diseases in Canada. Environ Int (2021) 157:106817. doi:10.1016/j.envint.2021.106817
89. Zou, H, Zhang, S, Cai, M, Qian, ZM, Zhang, Z, Chen, L, et al. Ambient Air Pollution Associated With Incidence and Progression Trajectory of Cardiometabolic Diseases: A Multi-State Analysis of a Prospective Cohort. Sci Total Environ (2023) 862(no pagination):160803. doi:10.1016/j.scitotenv.2022.160803
90. Byun, G, Choi, Y, Kim, S, and Lee, JT. Long-Term Exposure to Ambient Ozone and Mortality in a Population-Based Cohort of South Korea: Considering for an Alternative Exposure Time Metric. Environ Pollut (2022) 314:120300. doi:10.1016/j.envpol.2022.120300
91. Cakmak, S, Hebbern, C, Pinault, L, Lavigne, E, Vanos, J, Crouse, DL, et al. Associations Between Long-Term PM(2.5) and Ozone Exposure and Mortality in the Canadian Census Health and Environment Cohort (CANCHEC), by Spatial Synoptic Classification Zone. Environ Int (2018) 111:200–11. doi:10.1016/j.envint.2017.11.030
92. Di, Q, Wang, Y, Zanobetti, A, Wang, Y, Koutrakis, P, Choirat, C, et al. Air Pollution and Mortality in the Medicare Population. N Engl J Med (2017) 376(26):2513–22. doi:10.1056/NEJMoa1702747
93. Kazemiparkouhi, F, Eum, KD, Wang, B, Manjourides, J, and Suh, HH. Long-Term Ozone Exposures and Cause-Specific Mortality in a US Medicare Cohort. J Expo Sci Environ Epidemiol (2020) 30(4):650–8. doi:10.1038/s41370-019-0135-4
94. Yuan, Y, Wang, K, Sun, HZ, Zhan, Y, Yang, Z, Hu, K, et al. Excess Mortality Associated With High Ozone Exposure: A National Cohort Study in China. Environ Sci Ecotechnol (2023) 15:100241. doi:10.1016/j.ese.2023.100241
95. Zhang, Y, Yin, Z, Li, S, Zhang, JJ, Sun, HZ, Liu, K, et al. Ambient PM(2.5), Ozone and Mortality in Chinese Older Adults: A Nationwide Cohort Analysis (2005-2018). J Hazard Mater (2023) 454:131539. doi:10.1016/j.jhazmat.2023.131539
96. Abed, AAM, Demšar, U, Sullivan, F, and Kulu, H. Long-term Exposure to Air Pollution and Mortality in Scotland: A Register-Based Individual-Level Longitudinal Study. Environ Res (2023) 238(Pt 2):117223. doi:10.1016/j.envres.2023.117223
97. Jiang, Z, Zhang, S, Chen, K, Wu, Y, Zeng, P, and Wang, T. Long-Term Influence of Air Pollutants on Morbidity and All-Cause Mortality of Cardiometabolic Multi-Morbidity: A Cohort Analysis of the UK Biobank Participants. Environ Res (2023) 237(Pt 1):116873. doi:10.1016/j.envres.2023.116873
98. Li, Z-H, Wang, X-M, Xiang, J-X, Nan, Y, Chen, Y-J, Zhang, P-D, et al. Associations of Long-Term Joint Exposure to Various Ambient Air Pollutants with All-Cause and Cause-specific Mortality: Evidence From a Large Population-Based Cohort Study. Environ Sci Pollut Res Int (2023) 30(35):84357–67. doi:10.1007/s11356-023-28224-2
99. Peng, M, Zhang, F, Yuan, Y, Yang, Z, Wang, K, Wang, Y, et al. Long-term Ozone Exposure and All-Cause Mortality: Cohort Evidence in China and Global Heterogeneity by Region. Ecotoxicol Environ Saf (2023) 270:115843. doi:10.1016/j.ecoenv.2023.115843
100. Ripley, S, Gao, D, Pollitt, KJG, Lakey, PSJ, Shiraiwa, M, Hatzopoulou, M, et al. Within-City Spatial Variations in Long-Term Average Outdoor Oxidant Gas Concentrations and Cardiovascular Mortality: Effect Modification by Oxidative Potential in the Canadian Census Health and Environment Cohort. Environ Epidemiol (Philadelphia, Pa) (2023) 7(4):e257. doi:10.1097/EE9.0000000000000257
101. Wang, X, Ran, S, Xia, H, Shi, H, Wu, G, Zhang, Z, et al. Ambient Air Pollution Associated With Incident Asthma, Subsequent Cardiovascular Disease and Death: A Trajectory Analysis of a National Cohort. J Hazard Mater (2023) 460:132372. doi:10.1016/j.jhazmat.2023.132372
102. Xu, S, Marcon, A, Bertelsen, RJ, Benediktsdottir, B, Brandt, J, Engemann, K, et al. Long-term Exposure to Low-Level Air Pollution and Greenness and Mortality in Northern Europe. The Life-GAP Project. Environ Int (2023) 181:108257. doi:10.1016/j.envint.2023.108257
103. Boogaard, H, Atkinson, RW, Brook, JR, Chang, HH, Hoek, G, Hoffmann, B, et al. Evidence Synthesis of Observational Studies in Environmental Health: Lessons Learned From a Systematic Review on Traffic-Related Air Pollution. Environ Health Perspect (2023) 131(11):115002. doi:10.1289/ehp11532
104. National Institute of Environmental Health Sciences. Handbook for Conducting a Literature-Based Health Assessment Using OHAT Approach for Systematic Review and Evidence Integration. NC, United States: Office of Health Assessment and Translation, National Toxicology Program, National Institute of Environmental Health Sciences Research Triangle Park (2019). Available from: https://ntrl.ntis.gov/NTRL/dashboard/searchResults/titleDetail/PB2016102134.xhtml (Accessed: September 12, 2024).
105. Institute for Health Metrics and Evaluation (IHME). Burden of Proof. Seattle, WA: IHME, University of Washington (2024). Available from: https://vizhub.healthdata.org/burden-of-proof/ (Accessed: September 12, 2024).
106. Zheng, P, Afshin, A, Biryukov, S, Bisignano, C, Brauer, M, Bryazka, D, et al. The Burden of Proof Studies: Assessing the Evidence of Risk. Nat Med (2022) 28(10):2038–44. doi:10.1038/s41591-022-01973-2
107. Health Effect Institute. Systematic Review and Meta-Analysis of Selected Health Effects of Long-Term Exposure to Traffic-Related Air Pollution. Boston, MA, USA: HEI (2022).
108. Hoffmann, B, Boogaard, H, de Nazelle, A, Andersen, ZJ, Abramson, M, Brauer, M, et al. WHO Air Quality Guidelines 2021-Aiming for Healthier Air for All: A Joint Statement by Medical, Public Health, Scientific Societies and Patient Representative Organisations. Int J Public Health (2021) 66:1604465. doi:10.3389/ijph.2021.1604465
Keywords: long-term exposure, meta-analysis, mortality, nitrogen dioxide, ozone
Citation: Kasdagli M-I, Orellano P, Pérez Velasco R and Samoli E (2024) Long-Term Exposure to Nitrogen Dioxide and Ozone and Mortality: Update of the WHO Air Quality Guidelines Systematic Review and Meta-Analysis. Int J Public Health 69:1607676. doi: 10.3389/ijph.2024.1607676
Received: 20 June 2024; Accepted: 26 September 2024;
Published: 18 October 2024.
Edited by:
Nino Kuenzli, Swiss Tropical and Public Health Institute (Swiss TPH), SwitzerlandReviewed by:
Aaron Cohen, Health Effects Institute, United StatesNhung Nguyen, Hanoi University of Public Health, Vietnam
Copyright © World Health Organization 2024. Licensee Frontiers Media SA and Stiftung Swiss School of Public Health plus. This is an open access article distributed under the terms of the Creative Commons Attribution IGO License, which permits unrestricted use, adaptation (including derivative works), distribution, and reproduction in any medium, provided the original work is properly cited. In any reproduction or adaptation of this article there should not be any suggestion that WHO or this article endorse any specific organisation or products. The use of the WHO logo is not permitted. This notice should be preserved along with the article's original URL.
*Correspondence: Evangelia Samoli, ZXNhbW9saUBtZWQudW9hLmdy