- Department of Epidemiology and Public Health, Foch Hospital, Suresnes, France
Objective: The purpose of this study was to investigate the sex correlations of particulate matters (PM2.5, PM10, PM2.5–10), NO2 and NOx with ASCVD risk in the UK Biobank population.
Methods: Among 285,045 participants, pollutants were assessed and correlations between ASCVD risk were stratified by sex and estimated using multiple linear and logistic regressions adjusted for length of time at residence, education, income, physical activity, Townsend deprivation, alcohol, smocking pack years, BMI and rural/urban zone.
Results: Males presented higher ASCVD risk than females (8.63% vs. 2.65%, p < 0.001). In males PM2.5, PM10, NO2, and NOx each were associated with an increased ASCVD risk >7.5% in the adjusted logistic models, with ORs [95% CI] for a 10 μg/m3 increase were 2.17 [1.87–2.52], 1.15 [1.06–1.24], 1.06 [1.04–1.08] and 1.05 [1.04–1.06], respectively. In females, the ORs for a 10 μg/m3 increase were 1.55 [1.19–2.05], 1.22 [1.06–1.42], 1.07 [1.03–1.10], and 1.04 [1.02–1.05], respectively. No association was observed in both sexes between ASCVD risk and PM2.5–10.
Conclusion: Our findings may suggest the possible actions of air pollutants on ASCVD risk.
Introduction
Continuous exposure to air pollution over an extended period poses a significant public health threat [1]. Alarmingly, a significant proportion of Europeans living in urban areas, up to 30%, are exposed to air pollution levels exceeding European air-quality standards [2]. Air pollution is recognized as one of the most significant yet often overlooked threats to human health [3–6]. The World Health Organization (WHO) estimates that air pollution is responsible for 7.6% of global mortality [7].
Recently, the 2019 European Society of Cardiology (ESC) guidelines emphasized the impact of air pollution on cardiovascular diseases [8]. Numerous studies have established a direct link between long-term exposure to air pollution and an increased risk of cardiovascular events, including myocardial infarction, stroke, and cardiovascular mortality [9, 10]. Furthermore, controlled environment studies have demonstrated that exposure to particulate matter induces systemic vascular responses, oxidative stress, endothelial dysfunction, and plaque formation [11].
Atherosclerotic cardiovascular disease (ASCVD) is a leading cause of mortality and contributes significantly to healthcare costs worldwide [12]. However, few investigations have focused on individual air pollutants, such as particulate matter (PM2.5, PM10), nitrogen oxide (NOx), and nitrogen dioxide (NO2), and their correlations with ASCVD risk [11, 13, 14].
Numerous investigations have shown sex differences for ASCVD risk and cardiovascular diseases [15, 16], including coronary heart disease [17], carotid atherosclerosis [18], hypertension [19], atrial fibrillation ([20], pp. 1994–2016), cardiometabolic disorders [21] and stroke [22].
Furthermore, sex differences in air pollution-related cardiovascular diseases have been highlighted [23] with an impact on circulatory and respiratory mortality [24]. But there has been limited research into the relationship between air pollution and ASCVD risk, and particularly concerning sex differences. This connection is still incomplete [23, 25]. Thus, the purpose of this study was to investigate, firstly, the association of particulate matters, nitrogen dioxide and nitrogen oxide with 10 year ASCVD risk in the overall population, and secondly, in sex-stratification analyses these associations, in the UK Biobank population.
Methods
UK Biobank Population
Between 2006 and 2010, the UK Biobank recruited a total of 502,478 individuals aged 38–73 years from 22 cities across the UK, representing approximately 5.5% of the overall UK population. The participants provided comprehensive information through questionnaires, computer-assisted interviews, and a variety of physical and functional measurements. Additionally, samples of blood, urine, and saliva were collected for further analysis [26]. Further details about the cohort’s protocol can be found in relevant literature [27, 28].
Ethical Considerations
Prior to participation, all individuals provided electronic informed consent, and the UK Biobank obtained ethical approval from the North-West Multi-center Research Ethics Committee (MREC), which encompassed the entirety of the United Kingdom. The study adhered to the principles outlined in the Declaration of Helsinki and received approval from the Northwest—Haydock Research Ethics Committee (protocol code: 21/NW/0157, approval date: 21 June 2021). For details: [29].
Study Population
This study included a total of 399,067 participants from the UK Biobank who did not have missing data for ASCVD risk calculation and had no previous cardiovascular (CV) events. “CV diseases, including heart attack, angina, and stroke, were identified based on doctor diagnoses reported in the questionnaires” [30]. Moreover, this study also excluded 112,022 participants due to missing data for air pollutants (N = 33,774) and for other covariates (N = 78,248), resulting in a final analysis cohort of 287,045 participants (Figure 1).
Estimated 10 Years ASCVD Risk
To estimate the 10 years ASCVD risk, the study utilized the Pooled Cohort Equations (PCE) model [31, 32]. The PCE enables the calculation of sex- and race-specific estimates of the 10-year risk of ASCVD for adults between the ages of 40 and 79. A PCE score of 7.5% or higher indicates a high risk of ASCVD, while a score below 7.5% suggests a lower risk [33–36].
Air Pollution
The annual average concentrations of PM2.5, PM10, PM2.5–10, NO2, and NOx were estimated using the Land Use Regression (LUR) model, which was developed as part of the European Study of Cohorts for Air Pollution Effects project [37, 38]. The LUR model incorporated geospatial predictor variables derived from a Geographic Information System, including factors such as traffic, land use, and topography. These variables were used to calculate the spatial variations in annual average air pollutant concentrations.
For this study, the residential addresses provided by participants during the baseline visit in the UK Biobank were utilized to link the air pollution exposures of each participant to their records. The exposure data for PM2.5, PM10, PM2.5–10, NO2, and NOx were collected in 2010. In the case of NO2 and PM10, annual concentration data were available for multiple years (2005, 2006, 2007, and 2010 for NO2; 2007 and 2010 for PM10). The averaged values of NO2 and PM10 from these years were included in the analysis [39].
Covariates
Blood pressure measurements, including systolic and diastolic blood pressure (SBP, DBP), were recorded twice using an automated BP device (Omron 705 IT electronic blood pressure monitor; OMRON Healthcare Europe B.V. Kruisweg 577 2132 NA Hoofddorp) at the assessment center [40, 41].
The determination of diabetes status was based on several criteria: receiving anti-diabetic medication, being diagnosed with diabetes by a doctor, or having a fasting glucose concentration ≥7 mmol/L [41, 42]. Medications were identified through the question: “Do you regularly take any of the following medications?”. Self-reported information on medication use was thus collected. Detailed information on biological parameters can be found in the UK Biobank protocol [43]. Body mass index (BMI) was calculated as weight (in kg) divided by height squared (in meters) and categorized as high (BMI > 30 kg/m2), moderate (BMI between 25 and 30 kg/m2), and low (less than 25 kg/m2) [44].
Education level was classified into three categories according to the International Standard Classification for Education (ISCED): high (college or university degree (20 years of education), NVQ or HND or HNC or equivalent [19 years of education)], moderate [O levels/GCSEs or equivalent (10 years of education), A/AS levels or equivalent (13 years of education), other professional qualifications such as nursing or teaching, etc. (15 years of education)], and low [none of the aforementioned (7 years of education)] [45]. Income level was defined as high (greater than £52,000 per year), moderate (between £18,000 and £51,999 per year), and low (less than £18,000 per year) [36]. Townsend deprivation index scores were calculated using data aggregated for the participants’ residential postcodes [46].
Current tobacco smokers were identified as participants who responded “yes, on most or all days” or “yes, only occasionally” to the question “do you smoke tobacco now.” Smoking pack-years were calculated as the average number of packs smoked per day multiplied by the total number of years of smoking in lifetime. In the UK Biobank, the number of years of smoking is calculated by subtracting the age of starting smoking from the age smoking was stopped (or age at inclusion for current smokers) [47, 48].
Alcohol drinker status was determined based on participants’ responses regarding their alcohol consumption: “current,” “past,” or “never” [35, 49].
Participants’ physical activity levels were evaluated using a revised version of the International Physical Activity Questionnaire (IPAQ) [50], which they completed on a tablet computer during the initial assessment. The self-reported physical activity data were analyzed using the approach developed by Bradbury et al. [51]. The total MET-hours per week were categorized as low (<10.0), moderate (10.0–49.9), and high (≥50 excess MET-hours/week) based on the IPAQ guidelines [52].
Statistical Analysis
The characteristics of the study population were presented using median and 25th and 75th percentiles for continuous variables, and numbers and proportions for categorical variables. Group comparisons were conducted using either Student’s t-test or Mann-Whitney test for continuous variables, and Pearson’s χ2 test for categorical variables. The primary objective of the study was to examine the correlation between air pollutants and estimated 10 years ASCVD risk levels, as well as the risk of high cardiovascular (CV) risk (estimated 10 years ASCVD risk exceeding 7.5%). Multiple regression models were employed to analyze the relationship between air pollutants and estimated 10 years ASCVD risk. The results were expressed as beta coefficients (for each 10 μg/m3 increase) with 95% confidence intervals for the estimated 10 years ASCVD risk, and as odds ratios (OR) with 95% confidence intervals for the risk of exceeding 7.5% estimated 10 years ASCVD risk. The beta coefficients and OR were calculated per unit increase in 10 μg/m³ for air pollutants, and with the reference being the lowest quartile values of air pollutants (OR = 1.00). Firstly, the models were adjusted for various factors, including sex, length of time at residence, education, income level, physical activity, Townsend deprivation quintiles, alcohol consumption, smoking pack years, BMI categories, and rural/urban zone. Secondly, the models were stratified by sex. Analyses were stratified by sex through sex differences have been observed for ASCVD risk [15, 53], for air pollution impact on health [54, 55] and an interaction between sex and each air pollutants in this study (p for interaction, p < 0.001). Interactions were examined by including simultaneous sex, individual air pollutants and their interaction term. The adjustments were justified by their relationship with ASCVD risk and CV risk: education [56], income [30, 57], physical activity [58], smoking pack years [59], Townsend deprivation [30, 60] and BMI [61].
Multicollinearities between air pollutants have been investigated by spearman correlation coefficients. PM2.5, PM10, PM2.5–10, NO2 and NOX were considered independent in the different models due to their observed collinearities. Air pollutants were considered as continuous covariables and then, were categorized by quartiles (Q4 was the higher levels of air pollutants and Q1 the lowest quartile of levels). Non-linear relationship between air pollutants and CVD has been observed in previous studies [62, 63]. Thus, unadjusted non-linear splines of air pollutants with ASCVD risk were performed by polynomial quadratic regressions.
Statistics were performed using SAS software (version 9.4; SAS Institute, Carry, NC). A p-value < 0.05 was considered statistically significant.
Results
The characteristics of the overall participants are shown in Table 1. Males (n = 136,200, 47.4%) presented a higher mean estimated 10 years ASCVD risk compared to females (8.63% vs. 2.65%, p < 0.001), higher levels of high physical activity (23.49% vs. 19.39%, p < 0.001), of high educational level (37.68% vs. 35.35%, p < 0.001), of high income (30.60% vs. 24.82%, p < 0.001), of high BMI levels (23.75% vs. 21.91%, p < 0.001) but same proportion of rural residency (15.66% vs. 15.45%, p = 0.113) (Table 1). The mean (SD) concentrations of air pollutants were higher in males than in females, for PM10 (19.10 [18.00–20.35] μg/m3 vs. 19.14 [18.05–20.41] μg/m3, respectively, p < 0.001) and NO2 (27.80 [22.68–33.62] μg/m3 vs. 27.93 [22.77–33.79] μg/m3, respectively (p < 0.001) but not PM2.5, PM2.5–10 and NOx. The Spearman correlation coefficients among the five air pollutants are shown in Supplementary File S1. Splines of air pollutants with ASCVD risk are shown in Figure 2 and presented the non-linear relationships between air pollution and ASCVD risk.
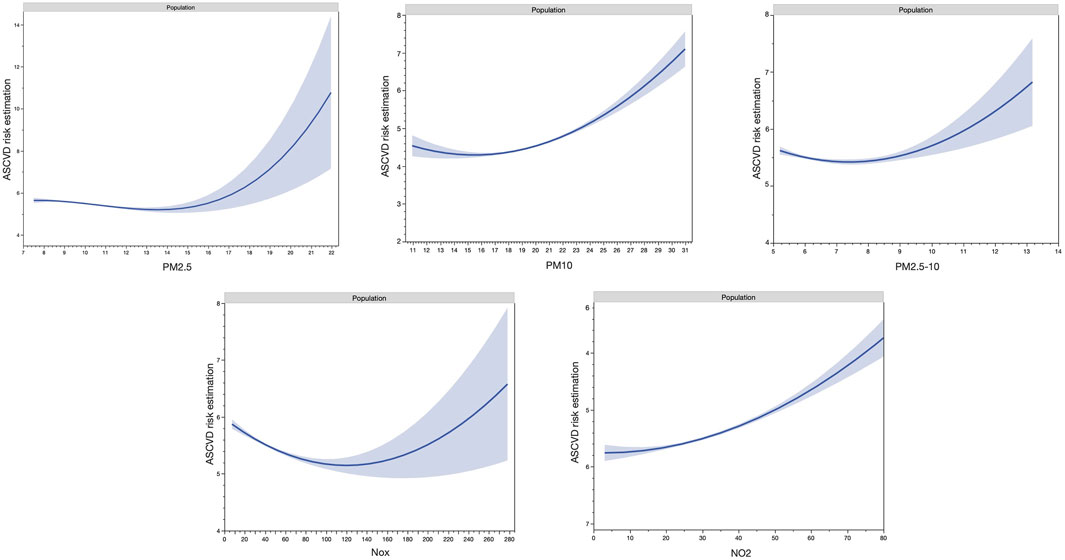
FIGURE 2. Non-linear splines of air pollutants with atherosclerotic cardiovascular disease risk (United Kingdom, 2021).
When considering the WHO 2005 recommendations [64] for annual toxicity threshold 46.04% of males and 46.55% of females were over the threshold for PM2.5 and 30.90% and 31.78%, respectively for PM10, and 10.98% and 11.45%, respectively for NO2. However, when considering the 2021 WHO recommendations [65], nearly 100% of the population showed over toxicity thresholds (Table 2).

TABLE 2. Proportion of males and females exposed to toxicity thresholds of Particle Matter 2.5, Particle Matter 10 and Nitrogen dioxide according to World Health Organization 2005 and [65] classifications (United Kingdom, 2021).
The associations between individual air pollutants and continuous ASCVD risk according to overall population and sex are shown in Table 3.
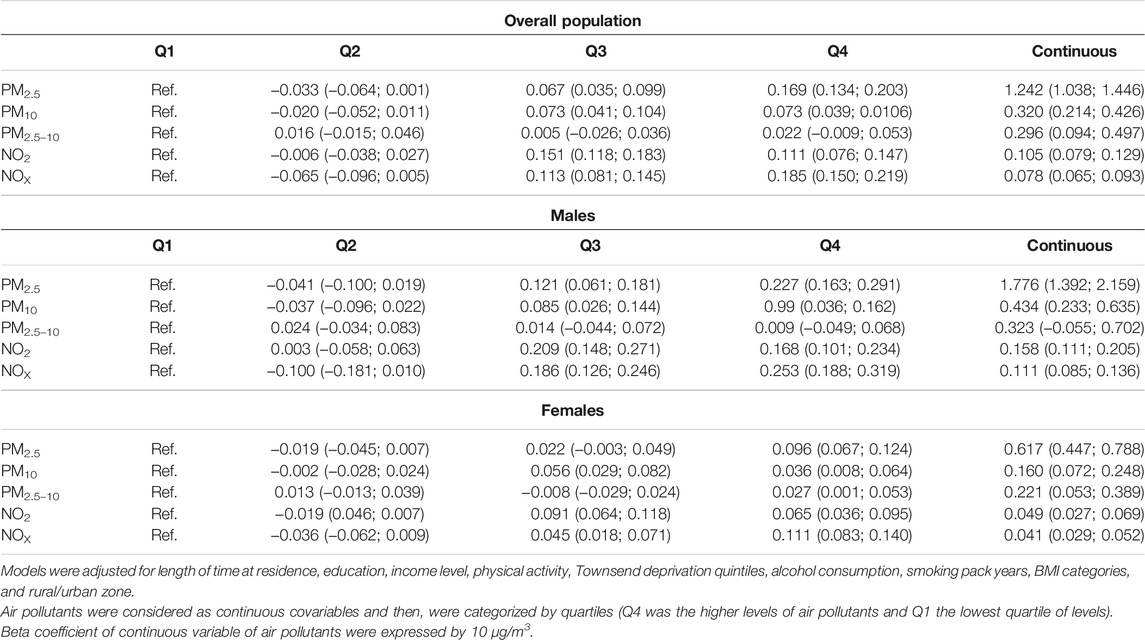
TABLE 3. Adjusted beta coefficients and 95% confidence interval for air pollution concentrations with continuous atherosclerotic cardiovascular disease risk (United Kingdom, 2021).
In overall population, PM2.5, PM10, NO2, and NOx each were associated with an increased continuous ASCVD risk in the adjusted linear models. No significant correlation was observed in males in the adjusted model between increased ASCVD risk and PM2.5–10, except when considering PM2.5–10 as a continuous variable.
In males, PM2.5, PM10, NO2, and NOx each were associated with an increased continuous ASCVD risk in the adjusted linear models. The beta coefficients (95% CI) of increase in continuous ASCVD risk for a 10 μg/m3 increase in PM2.5, PM10, NO2, and NOx were 1.776 (1.392; 2.159), 0.434 (0.233; 0.635), 0.158 (0.111; 0.205) and 0.111 (0.085; 0.136), respectively. No significant correlation was observed in males in the adjusted model between increased ASCVD risk and PM2.5–10 (Table 3).
Among females, both PM2.5, PM10, PM2.5–10, NO2, and NOx were associated with an increased continuous ASCVD risk in the adjusted linear models. The beta coefficients (95% CI) of increase in continuous ASCVD risk for a 10 μg/m3 increase in PM2.5, PM10, PM2.5–10, NO2, and NOx were 0.617 (0.447; 0.788), 0.160 (0.072; 0.248), 0.221 (0.053; 0.389), 0.049 (0.027; 0.069), and 0.041 (0.029; 0.052), respectively (Table 3).
When considering ASCVD risk >7.5%, in overall population, PM2.5, PM2.5–10, PM10, NO2, and NOx were all associated with an increased ASCVD risk >7.5% in the adjusted logistic models (Table 4).
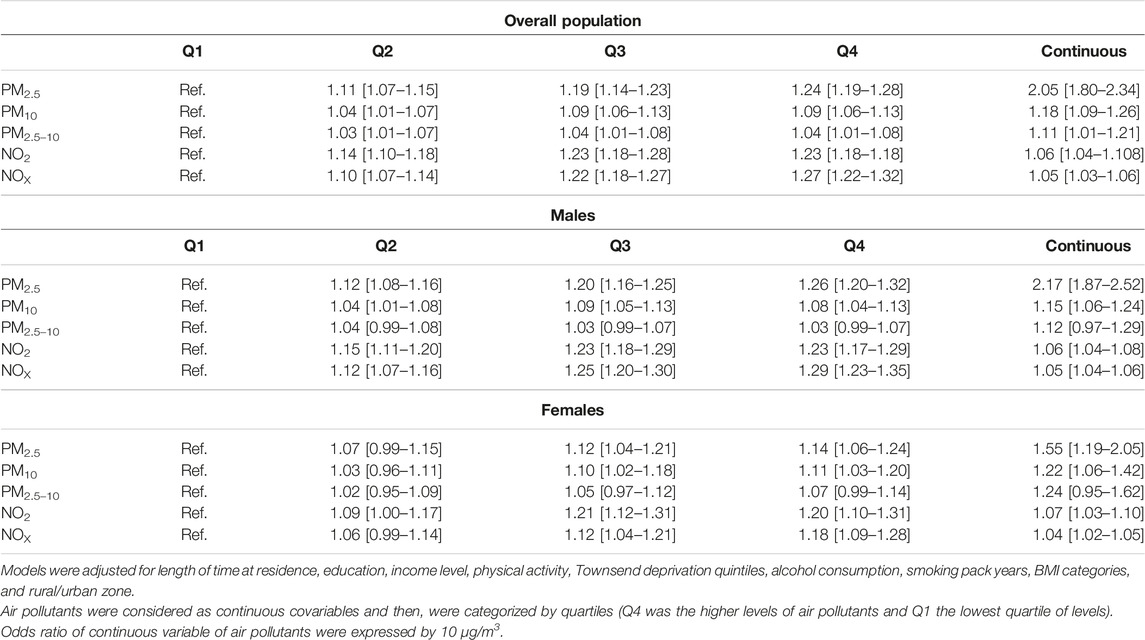
TABLE 4. Adjusted Odds ratio and 95% confidence interval for air pollution concentrations with atherosclerotic cardiovascular disease risk >7.5% (United Kingdom, 2021).
In males PM2.5, PM10, NO2, and NOx each were associated with an increased ASCVD risk>7.5% in the adjusted logistic models. The ORs [95% CI] of increase in continuous ASCVD risk for a 10 μg/m3 increase in PM2.5, PM10, NO2, and NOx were 2.17 [1.87–2.52], 1.15 [1.06–1.24], 1.06 [1.04–1.08] and 1.05 [1.04–1.06], respectively. No significant association was observed in males in the adjusted model between increased ASCVD risk and PM2.5–10 (Table 4).
Among females, both PM2.5, PM10, NO2, and NOx were associated with an increased ASCVD risk>7.5% in the adjusted logistic models. The ORs (95% CI) of increase in continuous ASCVD risk for a 10 μg/m3 increase in PM2.5, PM10, NO2, and NOx were 1.55 [1.19–2.05], 1.22 [1.06–1.42], 1.07 [1.03–1.10], and 1.04 [1.02–1.05], respectively. No significant association was observed in males in the adjusted model between increased ASCVD risk and PM2.5–10 (Table 4).
For both sexes, when considering the higher quartile groups compared to the lowest quartile, similar results were expressed (Tables 3, 4). When considering the ASCVD risk >7.5%, in males PM2.5, PM10, NO2, and NOx each were associated with an increased ASCVD risk >7.5% in the adjusted logistic models. The ORs [95% CI] for Q4 vs. Q1 were for PM2.5, PM10, NO2, and NOx, 1.26 [1.20–1.32], 1.08 [1.04–1.13], 1.23 [1.17–1.29], and 1.29 [1.23–1.35], respectively. And for females, the ORs [95% CI] for Q4 vs. Q1 were for PM2.5, PM10, NO2, and NOx, 1.12 [1.04–1.21], 1.10 [1.02–1.18], 1.21 [1.12–1.31] and 1.12 [1.04–1.21], respectively (Table 4). No significant association between PM2.5–10 and ASCVD risk was observed in both sexes.
Sensitive Analysis
When adding the “time of enrollment” in analyses, similar results were observed (Supplementary File S2).
Discussion
The primary finding of this study revealed a significant association between each individual air pollutants and an elevated risk of ASCVD in both males and females. Few sex differences were observed between individual air pollutants and ASCVD risk in this study. These results are consistent with previous studies in UK Biobank cohort [25, 39, 66–68].
Sex Differences
In 2022, a meta-analysis shown that CV events and mortality were increased by short exposure to PM2.5, in same average rate in both sexes [69]. But, when considering the long-term exposure to PM2.5 a sex differences was observed for ischemic heart disease [25]. Nevertheless, it remains controversial whether substantial differences would exist in air pollution with CV events between sexes. Numerous studies reported similar risk between sexes [70–72], while others reported differences in sexes [73, 74]. To date, no biological mechanism may explain the plausible differences observed in studies.
While, no differences in risk was observed between sexes, accurate health risk assessment remains essential to delivery optimal preventive medical care, and it is no longer acceptable to use a one-size-fits-all model of cardiovascular risk stratification which ignores sex differences [75]. Many tools or equations of cardiovascular risk assessment widely recommended by guidelines were developed based on sex-specific models along with different effect estimations even for the same risk factor [33, 76]. In forthcoming research concerning the interplay between air pollution and cardiovascular wellbeing, it is advisable to consistently present outcomes specific to sex. In the context of preventing and treating ischemic heart disease (IHD), data from both the US and China revealed a notable trend. It indicated that women were at a lower likelihood of receiving accurate diagnoses and receiving preventative care compared to men. This tendency might be connected to a perception of reduced risk among clinicians and patients towards women [77, 78].
Epidemiological Evidence
The findings of this study are consistent with prior studies examining the connection between air pollution and ASCVD risk [14, 79], heart failure [39], and ischemic heart disease [80]. Furthermore, air pollution has been associated with various other cardiovascular diseases, including cardiac arrhythmias and arrest, cerebrovascular disease, and venous thromboembolism [81, 82]. A study estimated that air pollution contributes to a global excess mortality of 8.8 million annually, resulting in a reduction of 2.9 years in life expectancy [83]. However, limited research has focused on different risk factors for ASCVD, such as blood lipid levels, hypertension, and diabetes [84–86].
Epidemiological studies have shown an association between prolonged exposure to PM2.5 and atherosclerosis, as measured by various indicators, such as carotid intima-media thickness, coronary, aortic calcifications, and ankle-brachial index [87]. Reduction in PM2.5 concentration has been associated to a decrease in the progression of intima-media carotid thickness [88]. The authors of the meta-analysis published in 2014 have found that prolonged exposure to particulate matter was associated with an increased incidence of coronary events [9]. Specifically, for every 5 μg/m3 increase in PM2.5 exposure, there was a 13% rise in non-fatal acute coronary events, and for every 10 μg/m3 increase in PM10 exposure, there was a 12% increase in coronary events. No relationship was found with other pollutants [9]. Another meta-analysis in 2015 demonstrated that PM2.5 and NO2 were significantly associated with diabetes [89]. Additionally, an increase in PM2.5 concentration by 10 μg/m3 has been linked to a 1–3 mmHg increase in systolic blood pressure a few days after exposure [90].
Moreover, the composition of PM stands as a pivotal aspect to deliberate, as certain discoveries highlight the heightened cardiovascular harm attributed to carbon-based particles originating from combustion-related sources like road traffic, fossil fuel usage, and wood combustion [91]. These combustion sources also contribute predominantly to the emission of nitrogen dioxide (NO2). A comprehensive analysis of the cardiovascular repercussions stemming from prolonged exposure to NO2 demonstrated a 13% surge in cardiovascular-related mortality following a mere 10 μg/m3 rise in annual NO2 concentrations [92]. The impact of ozone appears to exhibit a lesser magnitude, as some studies on long-term exposure imply a minor upswing in causes of cardiopulmonary mortality. Notably, this effect was only observable during warmer seasons and not in a year-round assessment. Numerous investigations have emphasized a robust link between extended exposure to air pollution and instances of acute myocardial infarction. In an expansive prospective study carried out across Europe, incremental yearly elevations of 10 μg/m3 in PM10 and 5 μg/m3 in PM2.5 were correlated with amplified risks of myocardial infarction, reaching 12% and 13% correspondingly [9].
Pathophysiological Mechanisms
Exposure to PM, particularly exposure to the polycyclic aromatic hydrocarbons present on the surface of PM originating from traffic, displayed a correlation with elevated levels of 8-hydroxy-2′-deoxyguanosine [93]. This biomarker is recognized for its reliability in indicating oxidative DNA damage in individuals subjected to such exposure, including individuals like traffic policemen, bus drivers, and garage workers [94]. Similarly, the occurrence of etheno-DNA adducts was double in diesel engine workers when compared to their non-diesel-exposed counterparts [95]. Various research studies have reported links between air pollution exposure and heightened plasma levels of oxidized low-density lipoprotein, homocysteine, as well as inflammatory markers and fibrinogen [96]. More recently, escalated concentrations of intercellular adhesion molecule-1 and vascular cell adhesion molecule-1 have been tied to exposure to air pollution [97]. The impact of air pollution exposure might facilitate the activation of inflammatory genes, as evidenced by studies revealing upregulation of antioxidant genes and a decline in overall DNA methylation [97]. Multiple investigations have affirmed that air pollution exposure triggers a robust oxidative stress response upon PM entry into the lungs. However, this pulmonary oxidative reaction is intensified through the activation of various enzymatic pathways, ultimately culminating in a systemic vascular oxidative stress reaction. After incubating endothelial cell cultures with serum obtained from voluntarily exposed subjects, the production of superoxide anions (reactive oxygen species [ROS]) was observed. This production followed a dose-response pattern directly linked to the quantity of inhaled PM2.5 [98]. The predominant role of ROS production was further confirmed by in vitro studies, revealing that superoxide dismutase reverses the adverse vascular effects subsequent to diesel exhaust exposure [99]. Studies have shown that the oxidative stress response was predominantly linked to the surface compounds coating diesel particles, including transition metals, polycyclic aromatic hydrocarbons, and quinones [99]. While oxidative stress primarily associates with particles, gaseous components such as NO2 are also contributors to ROS generation, forming peroxynitrite [93].
Exposure to diesel exhaust, in contrast to filtered air, resulted in impaired endothelium-dependent vasodilation and diminished endothelial NO bioavailability [100]. Endothelial dysfunction stands as an early indicator of atherosclerosis [101], with some initial functional consequences evident across various vascular beds. A decrease in patients ischemic threshold was observable, suggesting a detrimental influence of air pollution on myocardial blood flow regulation [102]. Air pollution favored the production of oxidized low-density lipoprotein and the release of other highly oxidized phospholipids [101]. These proatherogenic molecules permeated the subendothelial space, triggering the activation of endothelial cells. This activation was marked by the release of proinflammatory adhesion molecules, such as vascular cell adhesion molecule-1 and monocyte chemotactic protein-1 [101]. Furthermore, air pollution was linked to the impairment of antiatherogenic molecules like high-density lipoprotein [103]. A reduction in the antioxidant capacity of high-density lipoprotein was also observed in an animal study, with ultra-fine particles exhibiting a more pronounced effect compared to PM2.5 [101]. Consequently, exposure to air pollution, such as PM and NO2 [104], triggered vascular inflammation, accumulation of lipids in foam cells, and progression of arterial plaque, leading to increase the ASCVD risk [103].
Limitations
The study’s primary strength is its large sample size, which is drawn from the UK Biobank cohort. However, the cross-sectional design of the study limits the establishment of causal relationships and precludes determining reverse causation. Although the study had a response rate of only 5.5%, the robust sample size and high internal validity minimize the likelihood of participant bias influencing the observed associations [105, 106]. The generalizability of the study’s results to other age groups or ethnic populations may be limited since it focused exclusively on middle-aged participants from the UK. Nevertheless, the UK Biobank employed standardized protocols for data collection, ensuring replicability across all participants.
Several limitations should be acknowledged. Firstly, socio-economic data, medical history, and comorbidities were collected through self-reported questionnaires or physician assertion, which introduces the potential for bias. Additionally, the study’s temporal scope is restricted since data collection occurred only between 2006 and 2010. This study estimated the weights (regression coefficients) of air pollutants by treating each as a continuous variable. However, air pollutants were not linearly associated with ASCVD risk (Figure 2). Although examining non-linearity relations with the construction of quartiles of air pollutant may provide more information, the construction of the quartiles would not be sufficient. It is important to note that air pollution exposure may be either overestimated or underestimated, as the study did not gather data on pollution exposure in work environments, and only a single measurement of air pollution was available. The lack of address mobility is a major limitation in this study for the investigation of the relationship between air pollution exposure and ASCVD risk. Due to the high number of participants excluded due to missing data, the results observed should not be representative of the entire UK Biobank cohort. However, even though this study primarily focused on a specific population, it can still provide valuable insights as a reference when examining populations in other regions. Moreover, the results may not generalize to other populations, given that most participants in the UK Biobank were of European descent.
Conclusion
This study provides evidence that exposure to air pollutants, such as PM2.5, PM10, PM2.5–10, NO2 and NOx, throughout the year is associated with an increased risk of ASCVD in both males and females. These findings suggest the potential effects of air pollutants on ASCVD risk. They also highlight the need for implementing appropriate preventive health policies for populations affected by air pollution. Given the link between air pollution and ASCVD risk, it is crucial to gather information on individuals residing in polluted and urban areas or during periods of elevated air pollution levels.
Data Availability Statement
The data that support the findings of this study are available on request from the corresponding author. The data are not publicly available due to privacy or ethical restrictions.
Ethics Statement
All individuals provided electronic informed consent, and the UK Biobank obtained ethical approval from the North-West Multi-center Research Ethics Committee (MREC), which encompassed the entirety of the United Kingdom. The study adhered to the principles outlined in the Declaration of Helsinki and received approval from the Northwest—Haydock Research Ethics Committee (protocol code: 21/NW/0157, approval date: 21 June 2021). For details: [29]. The studies were conducted in accordance with the local legislation and institutional requirements. The participants provided their written informed consent to participate in this study.
Author Contributions
Conceptualization, methodology, formal analysis, and writing—original draft preparation, AV. The author has read and agreed to the published version of the manuscript.
Funding
This research has been conducted using the UK Biobank Resource under Application Number 55917.
Conflict of Interest
The author declares that they do not have any conflicts of interest.
Supplementary Material
The Supplementary Material for this article can be found online at: https://www.ssph-journal.org/articles/10.3389/ijph.2023.1606328/full#supplementary-material
References
1. Cohen, AJ, Brauer, M, Burnett, R, Anderson, HR, Frostad, J, Estep, K, et al. Estimates and 25-Year Trends of the Global Burden of Disease Attributable to Ambient Air Pollution: An Analysis of Data From the Global Burden of Diseases Study 2015. Lancet Lond Engl (2017) 389:1907–18. –1918. doi:10.1016/S0140-6736(17)30505-6
2. Visseren, FLJ, Mach, F, Smulders, YM, Carballo, D, Koskinas, KC, Bäck, M, et al. 2021 ESC Guidelines on Cardiovascular Disease Prevention in Clinical Practice. Eur Heart J (2021) 42:3227–337. doi:10.1093/eurheartj/ehab484
3. Kampa, M, and Castanas, E. Human Health Effects of Air Pollution. Environ Pollut Barking Essex (2008) 151:362–7. doi:10.1016/j.envpol.2007.06.012
4. Anderson, JO, Thundiyil, JG, and Stolbach, A. Clearing the Air: A Review of the Effects of Particulate Matter Air Pollution on Human Health. J Med Toxicol Off J Am Coll Med Toxicol (2012) 8:166–75. doi:10.1007/s13181-011-0203-1
5. Manisalidis, I, Stavropoulou, E, Stavropoulos, A, and Bezirtzoglou, E. Environmental and Health Impacts of Air Pollution: A Review. Front Public Health (2020) 8:14. doi:10.3389/fpubh.2020.00014
6. Fuller, R, Landrigan, PJ, Balakrishnan, K, Bathan, G, Bose-O'Reilly, S, Brauer, M, et al. Pollution and Health: A Progress Update. Lancet Planet Health (2022) 6:e535–e547. doi:10.1016/S2542-5196(22)00090-0
7. World Health Organization (WHO). WHO/Health topics/Air Pollution. Geneva, Switzerland: World Health Organization (2023).
8. Knuuti, J, Wijns, W, Saraste, A, Capodanno, D, Barbato, E, Funck-Brentano, C, et al. 2019 ESC Guidelines for the Diagnosis and Management of Chronic Coronary Syndromes. Eur Heart J (2020) 41:407–77. doi:10.1093/eurheartj/ehz425
9. Cesaroni, G, Forastiere, F, Stafoggia, M, Andersen, ZJ, Badaloni, C, Beelen, R, et al. Long Term Exposure to Ambient Air Pollution and Incidence of Acute Coronary Events: Prospective Cohort Study and Meta-Analysis in 11 European Cohorts From the ESCAPE Project. BMJ (2014) 348:f7412. doi:10.1136/bmj.f7412
10. Feigin, VL, Roth, GA, Naghavi, M, Parmar, P, Krishnamurthi, R, Chugh, S, et al. Global Burden of Stroke and Risk Factors in 188 Countries, During 1990-2013: A Systematic Analysis for the Global Burden of Disease Study 2013. Lancet Neurol (2016) 15:913–24. doi:10.1016/S1474-4422(16)30073-4
11. Bevan, GH, Al-Kindi, SG, Brook, RD, Münzel, T, and Rajagopalan, S. Ambient Air Pollution and Atherosclerosis: Insights Into Dose, Time, and Mechanisms. Arterioscler Thromb Vasc Biol (2021) 41:628–37. doi:10.1161/ATVBAHA.120.315219
12. Leening, MJG, Berry, JD, and Allen, NB. Lifetime Perspectives on Primary Prevention of Atherosclerotic Cardiovascular Disease. JAMA (2016) 315:1449–50. doi:10.1001/jama.2016.1654
13. Li, R, Hou, J, Tu, R, Liu, X, Zuo, T, Dong, X, et al. Associations of Mixture of Air Pollutants With Estimated 10-Year Atherosclerotic Cardiovascular Disease Risk Modified by Socio-Economic Status: The Henan Rural Cohort Study. Sci Total Environ (2021) 793:148542. doi:10.1016/j.scitotenv.2021.148542
14. Pan, M, Li, S, Tu, R, Li, R, Liu, X, Chen, R, et al. Associations of Solid Fuel Use and Ambient Air Pollution With Estimated 10-Year Atherosclerotic Cardiovascular Disease Risk. Environ Int (2021) 157:106865. doi:10.1016/j.envint.2021.106865
15. Okunrintemi, V, Valero-Elizondo, J, Patrick, B, Salami, J, Tibuakuu, M, Ahmad, S, et al. Gender Differences in Patient-Reported Outcomes Among Adults With Atherosclerotic Cardiovascular Disease. J Am Heart Assoc (2018) 7:e010498. doi:10.1161/JAHA.118.010498
16. Gao, Z, Chen, Z, Sun, A, and Deng, X. Gender Differences in Cardiovascular Disease. Med Nov Technol Devices (2019) 4:100025. doi:10.1016/j.medntd.2019.100025
17. Bots, SH, Peters, SAE, and Woodward, M. Sex Differences in Coronary Heart Disease and Stroke Mortality: A Global Assessment of the Effect of Ageing Between 1980 and 2010. BMJ Glob Health (2017) 2:e000298. doi:10.1136/bmjgh-2017-000298
18. van Dam-Nolen, DHK, van Egmond, NCM, Koudstaal, PJ, van der Lugt, A, and Bos, D. Sex Differences in Carotid Atherosclerosis: A Systematic Review and Meta-Analysis. Stroke (2023) 54:315–26. doi:10.1161/STROKEAHA.122.041046
19. Gerdts, E, Sudano, I, Brouwers, S, Borghi, C, Bruno, RM, Ceconi, C, et al. Sex Differences in Arterial Hypertension. Eur Heart J (2022) 43:4777–88. doi:10.1093/eurheartj/ehac470
20. Sharashova, E, Gerdts, E, Ball, J, Espnes, H, Jacobsen, BK, Kildal, S, et al. Sex-Specific Time Trends in Incident Atrial Fibrillation and the Contribution of Risk Factors: The Tromsø Study 1994-2016. Eur J Prev Cardiol (2023) 30:72–81. doi:10.1093/eurjpc/zwac234
21. Gerdts, E, and Regitz-Zagrosek, V. Sex Differences in Cardiometabolic Disorders. Nat Med (2019) 25:1657–66. doi:10.1038/s41591-019-0643-8
22. Bushnell, CD, Chaturvedi, S, Gage, KR, Herson, PS, Hurn, PD, Jiménez, MC, et al. Sex Differences in Stroke: Challenges and Opportunities. J Cereb Blood Flow Metab (2018) 38:2179–91. doi:10.1177/0271678X18793324
23. Liao, M, Braunstein, Z, and Rao, X. Sex Differences in Particulate Air Pollution-Related Cardiovascular Diseases: A Review of Human and Animal Evidence. Sci Total Environ (2023) 884:163803. doi:10.1016/j.scitotenv.2023.163803
24. Shin, HH, Maquiling, A, Thomson, EM, Park, IW, Stieb, DM, and Dehghani, P. Sex-Difference in Air Pollution-Related Acute Circulatory and Respiratory Mortality and Hospitalization. Sci Total Environ (2022) 806:150515. doi:10.1016/j.scitotenv.2021.150515
25. Zhang, J, Wang, X, Yan, M, Shan, A, Wang, C, Yang, X, et al. Sex Differences in Cardiovascular Risk Associated With Long-Term PM2.5 Exposure: A Systematic Review and Meta-Analysis of Cohort Studies. Front Public Health (2022) 10:802167. doi:10.3389/fpubh.2022.802167
26. Sudlow, C, Gallacher, J, Allen, N, Beral, V, Burton, P, Danesh, J, et al. UK Biobank: An Open Access Resource for Identifying the Causes of a Wide Range of Complex Diseases of Middle and Old Age. Plos Med (2015) 12:e1001779. doi:10.1371/journal.pmed.1001779
27. Bycroft, C, Freeman, C, Petkova, D, Band, G, Elliott, LT, Sharp, K, et al. The UK Biobank Resource With Deep Phenotyping and Genomic Data. Nature (2018) 562:203–9. doi:10.1038/s41586-018-0579-z
28. Vallée, A. Arterial Stiffness Determinants for Primary Cardiovascular Prevention Among Healthy Participants. J Clin Med (2022) 11:2512. doi:10.3390/jcm11092512
29. UK Biobank. Ethics (2023). Available at: https://www.ukbiobank.ac.uk/learn-more-about-uk-biobank/about-us/ethics (Accessed January, 2023).
30. Vallée, A. Association Between Socio-Economic Status and Estimated Atherosclerotic Cardiovascular Disease Risk: Results From a Middle-Aged Population-Based Study. Public Health (2023) 221:1–9. doi:10.1016/j.puhe.2023.05.014
31. Mozaffarian, D, Benjamin, EJ, Go, AS, Arnett, DK, Blaha, MJ, Cushman, M, et al. Heart Disease and Stroke Statistics-2015 Update: A Report From the American Heart Association. Circulation (2015) 131:e29–322. doi:10.1161/CIR.0000000000000152
32. Yadlowsky, S, Hayward, RA, Sussman, JB, McClelland, RL, Min, YI, and Basu, S. Clinical Implications of Revised Pooled Cohort Equations for Estimating Atherosclerotic Cardiovascular Disease Risk. Ann Intern Med (2018) 169:20–9. doi:10.7326/M17-3011
33. Goff, DC, Lloyd-Jones, DM, Bennett, G, Coady, S, D'Agostino, RB, Gibbons, R, et al. 2013 ACC/AHA Guideline on the Assessment of Cardiovascular Risk: A Report of the American College of Cardiology/American Heart Association Task Force on Practice Guidelines. J Am Coll Cardiol (2014) 63:2935–59. doi:10.1016/j.jacc.2013.11.005
34. Nonterah, EA, Boateng, D, Crowther, NJ, Klipstein-Grobusch, K, Oduro, AR, Agongo, G, et al. Carotid Atherosclerosis, Microalbuminuria, and Estimated 10-Year Atherosclerotic Cardiovascular Disease Risk in Sub-Saharan Africa. JAMA Netw Open (2022) 5:e227559. doi:10.1001/jamanetworkopen.2022.7559
35. Vallée, A. Association Between Social Isolation and Loneliness With Estimated Atherosclerotic Cardiovascular Disease Risk in a UK Biobank Population-Based Study. Int J Environ Res Public Health (2023) 20:2869. doi:10.3390/ijerph20042869
36. Vallée, A. Association Between Cannabis Use and Ten-Year Estimated Atherosclerotic Cardiovascular Disease Risk in a Middle-Aged Population Survey. Eur J Intern Med (2023) S0953-6205(23):69–76. –2. doi:10.1016/j.ejim.2023.02.020
37. Eeftens, M, Beelen, R, de Hoogh, K, Bellander, T, Cesaroni, G, Cirach, M, et al. Development of Land Use Regression Models for PM(2.5), PM(2.5) Absorbance, PM(10) and PM(Coarse) in 20 European Study Areas; Results of the ESCAPE Project. Environ Sci Technol (2012) 46:11195–205. doi:10.1021/es301948k
38. Beelen, R, Hoek, G, Vienneau, D, Eeftens, M, Dimakopoulou, K, Pedeli, X, et al. Development of NO2 and NOx Land Use Regression Models for Estimating Air Pollution Exposure in 36 Study Areas in Europe - The ESCAPE Project. Atmos Environ (2013) 72:10–23. doi:10.1016/j.atmosenv.2013.02.037
39. Wang, M, Zhou, T, Song, Y, Li, X, Ma, H, Hu, Y, et al. Joint Exposure to Various Ambient Air Pollutants and Incident Heart Failure: A Prospective Analysis in UK Biobank. Eur Heart J (2021) 42:1582–91. doi:10.1093/eurheartj/ehaa1031
40. UK Biobank. Arterial Pulse-Wave Velocity (2011). Available at: http://www.ukbiobank.ac.uk/ (Accessed April 15, 2011).
41. Vallée, A. Association Between Lipids and Arterial Stiffness for Primary Cardiovascular Prevention in a General Middle-Aged European Population. Front Cardiovasc Med (2022) 9:899841. doi:10.3389/fcvm.2022.899841
42. Emerging Risk Factors Collaboration Sarwar,, N, Gao, P, Seshasai, SRK, Gobin, R, Kaptoge, S, et al. Diabetes Mellitus, Fasting Blood Glucose Concentration, and Risk of Vascular Disease: A Collaborative Meta-Analysis of 102 Prospective Studies. Lancet Lond Engl (2010) 375:2215–22. doi:10.1016/S0140-6736(10)60484-9
43. UK Biobank. Biomarker Assay Quality Procedures: Approaches Used to Minimise Systematic and Random Errors (And the Wider Epidemiological Implications) (2019). Available at: https://biobank.ctsu.ox.ac.uk/crystal/crystal/docs/biomarker_issues.pdf (Accessed April 02, 2019).
44. Vallée, A. Association Between Serum Uric Acid and Arterial Stiffness in a Large-Aged 40-70 Years Old Population. J Clin Hypertens Greenwich Conn (2022) 24:885–97. doi:10.1111/jch.14527
45. Chadeau-Hyam, M, Bodinier, B, Vermeulen, R, Karimi, M, Zuber, V, Castagné, R, et al. Education, Biological Ageing, All-Cause and Cause-Specific Mortality and Morbidity: UK Biobank Cohort Study. EClinicalMedicine (2020) 29:100658. doi:10.1016/j.eclinm.2020.100658
47. Vallée, A. Associations Between Smoking and Alcohol Consumption With Blood Pressure in a Middle-Aged Population. Tob Induc Dis (2023) 21:61. doi:10.18332/tid/162440
48. Vallée, A. Association Between Tobacco Smoking and Alcohol Consumption With Arterial Stiffness. J Clin Hypertens Greenwich Conn (2023) 25:757–67. doi:10.1111/jch.14669
49. Vallée, A. Association Between Cannabis Use and Blood Pressure Levels According to Comorbidities and Socioeconomic Status. Sci Rep (2023) 13:2069. doi:10.1038/s41598-022-22841-6
50. Craig, CL, Marshall, AL, Sjöström, M, Bauman, AE, Booth, ML, Ainsworth, BE, et al. International Physical Activity Questionnaire: 12-Country Reliability and Validity. Med Sci Sports Exerc (2003) 35:1381–95. doi:10.1249/01.MSS.0000078924.61453.FB
51. Bradbury, KE, Guo, W, Cairns, BJ, Armstrong, MEG, and Key, TJ. Association Between Physical Activity and Body Fat Percentage, With Adjustment for BMI: A Large Cross-Sectional Analysis of UK Biobank. BMJ Open (2017) 7:e011843. doi:10.1136/bmjopen-2016-011843
52. O’Donnell, J, Smith-Byrne, K, Velardo, C, Conrad, N, Salimi-Khorshidi, G, Doherty, A, et al. Self-Reported and Objectively Measured Physical Activity in People With and Without Chronic Heart Failure: UK Biobank Analysis. Open Heart (2020) 7:e001099. doi:10.1136/openhrt-2019-001099
53. Kwak, S, Lee, H-J, Kim, S, Park, JB, and Kim, HK. Machine Learning Reveals Sex-Specific Associations Between Cardiovascular Risk Factors and Incident Atherosclerotic Cardiovascular Disease. Sci Rep (2023) 13:9364. doi:10.1038/s41598-023-36450-4
54. Clougherty, JE. A Growing Role for Gender Analysis in Air Pollution Epidemiology. Environ Health Perspect (2010) 118:167–76. doi:10.1289/ehp.0900994
55. Kuźma, Ł, Struniawski, K, Pogorzelski, S, Bachórzewska-Gajewska, H, and Dobrzycki, S. Gender Differences in Association Between Air Pollution and Daily Mortality in the Capital of the Green Lungs of Poland-Population-Based Study With 2,953,000 Person-Years of Follow-Up. J Clin Med (2020) 9:2351. doi:10.3390/jcm9082351
56. Henderson, K, Kaufman, B, Rotter, JS, Stearns, S, Sueta, CAA, Foraker, R, et al. Socioeconomic Status and Modification of Atherosclerotic Cardiovascular Disease Risk Prediction: Epidemiological Analysis Using Data From the Atherosclerosis Risk in Communities Study. BMJ Open (2022) 12:e058777. doi:10.1136/bmjopen-2021-058777
57. Nahodyl, L, Vilchez, L, Mesa, RA, Tremblay, J, and Elfassy, T. Abstract P232: Associations Between Income and Education With ASCVD Risk, NHANES, 1999-2018. Circulation (2022) 145:AP232. –AP232. doi:10.1161/circ.145.suppl_1.P232
58. Rao, P, Belanger, MJ, and Robbins, JM. Exercise, Physical Activity, and Cardiometabolic Health: Insights Into the Prevention and Treatment of Cardiometabolic Diseases. Cardiol Rev (2022) 30:167–78. doi:10.1097/CRD.0000000000000416
59. Duncan, MS, Greevy, RA, Tindle, HA, Vasan, RS, Lipworth, L, Aldrich, MC, et al. Inclusion of Smoking Data in Cardiovascular Disease Risk Estimation. JAMA Cardiol (2022) 7:195–203. doi:10.1001/jamacardio.2021.4990
60. Foster, HME, Celis-Morales, CA, Nicholl, BI, Petermann-Rocha, F, Pell, JP, Gill, JMR, et al. The Effect of Socioeconomic Deprivation on the Association Between an Extended Measurement of Unhealthy Lifestyle Factors and Health Outcomes: A Prospective Analysis of the UK Biobank Cohort. Lancet Public Health (2018) 3:e576–85. e585. doi:10.1016/S2468-2667(18)30200-7
61. Khera, R, Pandey, A, Ayers, CR, Carnethon, MR, Greenland, P, Ndumele, CE, et al. Performance of the Pooled Cohort Equations to Estimate Atherosclerotic Cardiovascular Disease Risk by Body Mass Index. JAMA Netw Open (2020) 3:e2023242. doi:10.1001/jamanetworkopen.2020.23242
62. Kriit, HK, Andersson, EM, Carlsen, HK, Andersson, N, Ljungman, PLS, Pershagen, G, et al. Using Distributed Lag Non-Linear Models to Estimate Exposure Lag-Response Associations Between Long-Term Air Pollution Exposure and Incidence of Cardiovascular Disease. Int J Environ Res Public Health (2022) 19:2630. doi:10.3390/ijerph19052630
63. Lin, Y-C, Shih, H-S, and Lai, C-Y. Long-Term Nonlinear Relationship Between PM2.5 and Ten Leading Causes of Death. Environ Geochem Health (2022) 44:3967–90. doi:10.1007/s10653-021-01136-1
64. World Health OrganizationRegional Office for Europe. Air Quality Guidelines: Global Update 2005: Particulate Matter, Ozone, Nitrogen Dioxide and Sulfur Dioxide. Geneva, Switzerland: World Health Organization (2006).
65. World Health Organization. WHO Global Air Quality Guidelines: Particulate Matter (PM2.5 and PM10), Ozone, Nitrogen Dioxide, Sulfur Dioxide and Carbon Monoxide. Geneva, Switzerland: World Health Organization (2021).
66. Cai, Y, Hodgson, S, Blangiardo, M, Gulliver, J, Morley, D, Fecht, D, et al. Road Traffic Noise, Air Pollution and Incident Cardiovascular Disease: A Joint Analysis of the HUNT, EPIC-Oxford and UK Biobank Cohorts. Environ Int (2018) 114:191–201. doi:10.1016/j.envint.2018.02.048
67. Zhang, S, Qian, ZM, Chen, L, Zhao, X, Cai, M, Wang, C, et al. Exposure to Air Pollution During Pre-Hypertension and Subsequent Hypertension, Cardiovascular Disease, and Death: A Trajectory Analysis of the UK Biobank Cohort. Environ Health Perspect (2023) 131:17008. doi:10.1289/EHP10967
68. Ma, Y, Li, D, Xie, J, Hu, Y, Su, B, and Tian, Y. Exposure to Various Ambient Air Pollutants and 9 Cardiovascular Conditions Among Individuals With Diabetes: A Prospective Analysis of the UK Biobank. Atherosclerosis (2023) 369:1–8. doi:10.1016/j.atherosclerosis.2023.02.002
69. Heo, S, Son, J-Y, Lim, CC, Fong, KC, Choi, HM, Hernandez-Ramirez, RU, et al. Effect Modification by Sex for Associations of fine Particulate Matter (PM2.5) With Cardiovascular Mortality, Hospitalization, and Emergency Room Visits: Systematic Review and Meta-Analysis. Environ Res Lett ERL Web Site (2022) 17:053006. doi:10.1088/1748-9326/ac6cfb
70. Wong, CM, Lai, HK, Tsang, H, Thach, TQ, Thomas, GN, Lam, KBH, et al. Satellite-Based Estimates of Long-Term Exposure to Fine Particles and Association With Mortality in Elderly Hong Kong Residents. Environ Health Perspect (2015) 123:1167–72. doi:10.1289/ehp.1408264
71. Bai, L, Shin, S, Burnett, RT, Kwong, JC, Hystad, P, van Donkelaar, A, et al. Exposure to Ambient Air Pollution and the Incidence of Congestive Heart Failure and Acute Myocardial Infarction: A Population-Based Study of 5.1 Million Canadian Adults Living in Ontario. Environ Int (2019) 132:105004. doi:10.1016/j.envint.2019.105004
72. Li, J, Liu, F, Liang, F, Huang, K, Yang, X, Xiao, Q, et al. Long-Term Effects of High Exposure to Ambient Fine Particulate Matter on Coronary Heart Disease Incidence: A Population-Based Chinese Cohort Study. Environ Sci Technol (2020) 54:6812–21. doi:10.1021/acs.est.9b06663
73. Wang, B, Eum, K-D, Kazemiparkouhi, F, Li, C, Manjourides, J, Pavlu, V, et al. The Impact of Long-Term PM2.5 Exposure on Specific Causes of Death: Exposure-Response Curves and Effect Modification Among 53 Million U.S. Medicare Beneficiaries. Environ Health Glob Access Sci Source (2020) 19:20. doi:10.1186/s12940-020-00575-0
74. Hystad, P, Larkin, A, Rangarajan, S, AlHabib, KF, Avezum, Á, Calik, KBT, et al. Associations of Outdoor Fine Particulate Air Pollution and Cardiovascular Disease in 157 436 Individuals From 21 High-Income, Middle-Income, and Low-Income Countries (PURE): A Prospective Cohort Study. Lancet Planet Health (2020) 4:e235–45. doi:10.1016/S2542-5196(20)30103-0
75. Agarwala, A, Michos, ED, Samad, Z, Ballantyne, CM, and Virani, SS. The Use of Sex-Specific Factors in the Assessment of Women’s Cardiovascular Risk. Circulation (2020) 141:592–9. doi:10.1161/CIRCULATIONAHA.119.043429
76. Piepoli, MF, Hoes, AW, Agewall, S, Albus, C, Brotons, C, Catapano, AL, et al. 2016 European Guidelines on Cardiovascular Disease Prevention in Clinical Practice: The Sixth Joint Task Force of the European Society of Cardiology and Other Societies on Cardiovascular Disease Prevention in Clinical Practice (Constituted by Representatives of 10 Societies and by Invited experts) Developed With the Special Contribution of the European Association for Cardiovascular Prevention & Rehabilitation (EACPR). Eur Heart J (2016) 37:2315–81. doi:10.1093/eurheartj/ehw106
77. Mosca, L, Linfante, AH, Benjamin, EJ, Berra, K, Hayes, SN, Walsh, BW, et al. National Study of Physician Awareness and Adherence to Cardiovascular Disease Prevention Guidelines. Circulation (2005) 111:499–510. doi:10.1161/01.CIR.0000154568.43333.82
78. Hao, Y, Liu, J, Liu, J, Yang, N, Smith, SC, Huo, Y, et al. Sex Differences in In-Hospital Management and Outcomes of Patients With Acute Coronary Syndrome. Circulation (2019) 139:1776–85. doi:10.1161/CIRCULATIONAHA.118.037655
79. Tu, R, Hou, J, Liu, X, Li, R, Dong, X, Pan, M, et al. Physical Activity Attenuated Association of Air Pollution With Estimated 10-Year Atherosclerotic Cardiovascular Disease Risk in a Large Rural Chinese Adult Population: A Cross-Sectional Study. Environ Int (2020) 140:105819. doi:10.1016/j.envint.2020.105819
80. Thurston, GD, Burnett, RT, Turner, MC, Shi, Y, Krewski, D, Lall, R, et al. Ischemic Heart Disease Mortality and Long-Term Exposure to Source-Related Components of U.S. Fine Particle Air Pollution. Environ Health Perspect (2016) 124:785–94. doi:10.1289/ehp.1509777
81. Shah, ASV, Langrish, JP, Nair, H, McAllister, DA, Hunter, AL, Donaldson, K, et al. Global Association of Air Pollution and Heart Failure: A Systematic Review and Meta-Analysis. Lancet Lond Engl (2013) 382:1039–48. doi:10.1016/S0140-6736(13)60898-3
82. Newby, DE, Mannucci, PM, Tell, GS, Baccarelli, AA, Brook, RD, Donaldson, K, et al. Expert Position Paper on Air Pollution and Cardiovascular Disease. Eur Heart J (2015) 36:83–93b. doi:10.1093/eurheartj/ehu458
83. Lelieveld, J, Pozzer, A, Pöschl, U, Fnais, M, Haines, A, and Münzel, T. Loss of Life Expectancy From Air Pollution Compared to Other Risk Factors: A Worldwide Perspective. Cardiovasc Res (2020) 116:1910–7. doi:10.1093/cvr/cvaa025
84. Cai, Y, Hansell, AL, Blangiardo, M, Burton, PR, de Hoogh, K, et al. BioSHaRE Long-Term Exposure to Road Traffic Noise, Ambient Air Pollution, and Cardiovascular Risk Factors in the HUNT and Lifelines Cohorts. Eur Heart J (2017) 38:2290–6. doi:10.1093/eurheartj/ehx263
85. Li, N, Chen, G, Liu, F, Mao, S, Liu, Y, Hou, Y, et al. Associations of Long-Term Exposure to Ambient PM1 With Hypertension and Blood Pressure in Rural Chinese Population: The Henan Rural Cohort Study. Environ Int (2019) 128:95–102. doi:10.1016/j.envint.2019.04.037
86. Liu, F, Guo, Y, Liu, Y, Chen, G, Wang, Y, Xue, X, et al. Associations of Long-Term Exposure to PM1, PM2.5, NO2 With Type 2 Diabetes Mellitus Prevalence and Fasting Blood Glucose Levels in Chinese Rural Populations. Environ Int (2019) 133:105213. doi:10.1016/j.envint.2019.105213
87. Muscente, F, and de Caterina, R. Pollution and Coronary Risk: How Much Does It Matter? Eur Heart J Suppl J Eur Soc Cardiol (2022) 24:I76–I80. doi:10.1093/eurheartjsupp/suac076
88. Adar, SD, Sheppard, L, Vedal, S, Polak, JF, Sampson, PD, Diez Roux, AV, et al. Fine Particulate Air Pollution and the Progression of Carotid Intima-Medial Thickness: A Prospective Cohort Study From the Multi-Ethnic Study of Atherosclerosis and Air Pollution. Plos Med (2013) 10:e1001430. doi:10.1371/journal.pmed.1001430
89. Eze, IC, Hemkens, LG, Bucher, HC, Hoffmann, B, Schindler, C, Künzli, N, et al. Association Between Ambient Air Pollution and Diabetes Mellitus in Europe and North America: Systematic Review and Meta-Analysis. Environ Health Perspect (2015) 123:381–9. doi:10.1289/ehp.1307823
90. Haberzettl, P, Conklin, DJ, Abplanalp, WT, Bhatnagar, A, and O'Toole, TE. Inhalation of Fine Particulate Matter Impairs Endothelial Progenitor Cell Function via Pulmonary Oxidative Stress. Arterioscler Thromb Vasc Biol (2018) 38:131–42. doi:10.1161/ATVBAHA.117.309971
91. Laden, F, Neas, LM, Dockery, DW, and Schwartz, J. Association of Fine Particulate Matter From Different Sources With Daily Mortality in Six U.S. Cities. Environ Health Perspect (2000) 108:941–7. doi:10.1289/ehp.00108941
92. Faustini, A, Rapp, R, and Forastiere, F. Nitrogen Dioxide and Mortality: Review and Meta-Analysis of Long-Term Studies. Eur Respir J (2014) 44:744–53. doi:10.1183/09031936.00114713
93. Bourdrel, T, Bind, M-A, Béjot, Y, Morel, O, and Argacha, JF. Cardiovascular Effects of Air Pollution. Arch Cardiovasc Dis (2017) 110:634–42. doi:10.1016/j.acvd.2017.05.003
94. Bagryantseva, Y, Novotna, B, Rossner, P, Chvatalova, I, Milcova, A, Svecova, V, et al. Oxidative Damage to Biological Macromolecules in Prague Bus Drivers and Garagemen: Impact of Air Pollution and Genetic Polymorphisms. Toxicol Lett (2010) 199:60–8. doi:10.1016/j.toxlet.2010.08.007
95. Shen, M, Bin, P, Li, H, Zhang, X, Sun, X, Duan, H, et al. Increased Levels of Etheno-DNA Adducts and Genotoxicity Biomarkers of Long-Term Exposure to Pure Diesel Engine Exhaust. Sci Total Environ (2016) 543:267–73. doi:10.1016/j.scitotenv.2015.10.165
96. Jacobs, L, Emmerechts, J, Hoylaerts, MF, Mathieu, C, Hoet, PH, Nemery, B, et al. Traffic Air Pollution and Oxidized LDL. PLoS ONE (2011) 6:e16200. doi:10.1371/journal.pone.0016200
97. Bind, M-A, Baccarelli, A, Zanobetti, A, Tarantini, L, Suh, H, Vokonas, P, et al. Air Pollution and Markers of Coagulation, Inflammation, and Endothelial Function: Associations and Epigene-Environment Interactions in an Elderly Cohort. Epidemiol Camb Mass (2012) 23:332–40. doi:10.1097/EDE.0b013e31824523f0
98. Wauters, A, Dreyfuss, C, Pochet, S, Hendrick, P, Berkenboom, G, van de Borne, P, et al. Acute Exposure to Diesel Exhaust Impairs Nitric Oxide-Mediated Endothelial Vasomotor Function by Increasing Endothelial Oxidative Stress. Hypertens Dallas Tex (2013) 62:352–8. doi:10.1161/HYPERTENSIONAHA.111.00991
99. Mills, NL, Miller, MR, Lucking, AJ, Beveridge, J, Flint, L, Boere, AJF, et al. Combustion-Derived Nanoparticulate Induces the Adverse Vascular Effects of Diesel Exhaust Inhalation. Eur Heart J (2011) 32:2660–71. doi:10.1093/eurheartj/ehr195
100. Mills, NL, Törnqvist, H, Robinson, SD, Gonzalez, M, Darnley, K, MacNee, W, et al. Diesel Exhaust Inhalation Causes Vascular Dysfunction and Impaired Endogenous Fibrinolysis. Circulation (2005) 112:3930–6. doi:10.1161/CIRCULATIONAHA.105.588962
101. Araujo, JA. Particulate Air Pollution, Systemic Oxidative Stress, Inflammation, and Atherosclerosis. Air Qual Atmosphere Health (2011) 4:79–93. doi:10.1007/s11869-010-0101-8
102. Mills, NL, Törnqvist, H, Gonzalez, MC, Vink, E, Robinson, SD, Söderberg, S, et al. Ischemic and Thrombotic Effects of Dilute Diesel-Exhaust Inhalation in Men with Coronary Heart Disease. N Engl J Med (2007) 357:1075–82. doi:10.1056/NEJMoa066314
103. Brook, RD, Rajagopalan, S, Pope, CA, Brook, JR, Bhatnagar, A, Diez-Roux, AV, et al. Particulate Matter Air Pollution and Cardiovascular Disease: An Update to the Scientific Statement From the American Heart Association. Circulation (2010) 121:2331–78. doi:10.1161/CIR.0b013e3181dbece1
104. Brunekreef, B, Strak, M, Chen, J, Andersen, ZJ, Atkinson, R, Bauwelinck, M, et al. Mortality and Morbidity Effects of Long-Term Exposure to Low-Level PM2.5, BC, NO2, and O3: An Analysis of European Cohorts in the ELAPSE Project. Res Rep Health Eff Inst (2021) 2021:1–127.
105. Richiardi, L, Pizzi, C, and Pearce, N. Commentary: Representativeness Is Usually Not Necessary and Often Should Be Avoided. Int J Epidemiol (2013) 42:1018–22. doi:10.1093/ije/dyt103
Keywords: air pollution, atherosclerosis, cardiovascular risk, sex, public health
Citation: Vallée A (2023) Sex Associations Between Air Pollution and Estimated Atherosclerotic Cardiovascular Disease Risk Determination. Int J Public Health 68:1606328. doi: 10.3389/ijph.2023.1606328
Received: 20 June 2023; Accepted: 19 September 2023;
Published: 28 September 2023.
Edited by:
Heresh Amini, Icahn School of Medicine at Mount Sinai, United StatesReviewed by:
Jiawei Zhang, University of Copenhagen, DenmarkPablo Knobel, Icahn School of Medicine at Mount Sinai, United States
Copyright © 2023 Vallée. This is an open-access article distributed under the terms of the Creative Commons Attribution License (CC BY). The use, distribution or reproduction in other forums is permitted, provided the original author(s) and the copyright owner(s) are credited and that the original publication in this journal is cited, in accordance with accepted academic practice. No use, distribution or reproduction is permitted which does not comply with these terms.
*Correspondence: Alexandre Vallée, YWwudmFsbGVlQGhvcGl0YWwtZm9jaC5jb20=