- 1Department of Hematology, Taizhou Hospital of Zhejiang Province Affiliated to Wenzhou Medical University, Linhai, Zhejiang, China
- 2School of Public Health, The University of Hong Kong Li Ka Shing Faculty of Medicine, Pokfulam, Hong Kong, Hong Kong SAR, China
- 3Evidence-Based Medicine Center, Taizhou Hospital of Zhejiang Province Affiliated to Wenzhou Medical University, Linhai, Zhejiang, China
Objectives: We aimed to assess the association between rapid antigen detection tests and real-time reverse transcription-polymerase chain reaction assay for severe acute respiratory syndrome coronavirus 2.
Methods: We searched PubMed, Cochrane Library, EMBASE, and the Web of Science from their inception to 31 May 2023. A random-effects meta-analysis was used to estimate false positives in the RADTs group, relative to those in the RT-PCR group, and subgroup analyses were conducted based on the different Ct value cut-offs (<40 or ≥40). We performed this study in accordance with the guidelines outlined in the Preferred Reporting Items for Systematic Reviews and Meta-Analyses (PRISMA).
Results: Fifty-one studies were included and considered to be of moderate quality. We found a satisfactory overall false positive rate (0.01, 95% CI: 0.00–0.01) for the RADTs compared to RT-PCR. In the stratified analysis, we also found that the false positive rates of the RADTs did not increase when Ct values of RT-PCR (Ct < 40, 0.01, 95% CI: 0.00–0.01; Ct ≥ 40, 0.01, 95% CI: 0.00–0.01).
Conclusion: In conclusion, the best available evidence supports an association between RADTs and RT-PCR. When Ct-values were analyzed using cut-off <40 or ≥40, this resulted in an estimated false positive rate of only 1%.
Introduction
COVID-19 remains an ongoing global pandemic, and the return to pre-pandemic normalcy is still projected to be unlikely in the short run [1]. It appears that the new linages are more capable of resisting natural or vaccine-elicited immunity [2, 3]. Hence, new linages may be responsible for major re-infections and mass vaccine breakthroughs, posing overwhelming pressure on health systems [2, 3]. Frequent early testing of infectious persons, in combination with contact tracing and isolation, are key factors that mitigate transmission [4]. However, false negatives, false positives, and other inaccurate results make it even more challenging for governing authorities to set effective control strategies and make timely medical decisions [5]. Therefore, policymakers have focused on these problems and explored some cost-effective tests [6, 7].
To improve sensitivity and specificity, reverse transcription-polymerase chain reaction (RT-PCR) has been the standard method for detecting severe acute respiratory syndrome coronavirus 2 (SARS-CoV-2) since the beginning of the pandemic [8]. However, RT-PCR testing needs a remarkably long turnaround time and relies heavily on sophisticated equipment and highly trained personnel. This limits its application in mass-oriented testing campaigns [9]. Thus, the World Health Organization (WHO) recommended simple and rapid antigen detection tests (RADTs) in communities to serve the purpose of detection and contact tracing, as well as outbreak investigations [10]. This technique does not need trained experts and professional instruments and can offer results within 15 min, making it possible to identify those potentially infected with COVID-19 on time [11]. However, the WHO suggests a minimum of 80% sensitivity and 97% specificity to adopt RADTs [12], since their performance is inconsistent in diverse settings according to published research [13].
Moreover, data on the performance of self-testing with RADTs compared to RT-PCR detection of SARS-CoV-2 RNA is very limited. Previous studies have demonstrated the accuracy of RADTs [13, 14], while few have explored the relationship between RADTs and cycle threshold (Ct) value cut-offs, allowing a gap for further research. Ct values vary in different areas and are dynamically adjusted. From a clinical viewpoint, there is no consistent standardization between laboratories and assays. Thus, we aimed to explore the association between RADTs and RT-PCR for SARS-CoV-2 based on the disparity of the Ct value.
Methods
Literature Search
We conducted the meta-analysis following the Preferred Reporting Items for Systematic Reviews and Meta-Analyses (PRISMA) guideline. We performed a systematic search of the databases PubMed, Cochrane Library, EMBASE, and the Web of Science from their inception to 31 May 2023. The main search terms were “[(COVID-19 OR SARS-CoV-2 OR Coronavirus disease 2019 OR Novel coronavirus) AND (Rapid diagnosis* OR Rapid detection OR Rapid antigen test* OR Antigen assay) AND (false-positive OR false-positivity OR specificity OR accuracy)] AND (cycle threshold OR Ct)] (Table 1). We also searched the previous reviews for relevant studies. We registered this systematic review on PROSPERO (registration number: CRD42022351138). No language restrictions were applied.
Inclusion Criteria and Exclusion Criteria
We included studies evaluating the specificity or false-positivity of commercially available RADTs for diagnosis of SARS-CoV-2 infection, against RT-PCR as a reference standard. Cohort studies, nested cohort studies, and case-control or cross-sectional studies as well as randomized studies were considered. Studies carried out in various locations, targeted at individuals of any age despite presence of symptoms were pooled in our study. Studies without reporting a Ct value cut-off were excluded.
Study Selection and Data Extraction
Two authors (Y-PY and ZJ) independently scanned the titles and abstracts of the search results in Endnote X9 to retrieve relevant records and obtained a full-text review of those eligible articles that met our inclusion criteria. Then, a third author (T-HT) was invited to settle any conflicts or disputes. Figure 1 illustrates the flowchart of the screening process. Based on the previously defined excluded criteria, 51 papers written in the English language were included in the final meta-analysis.
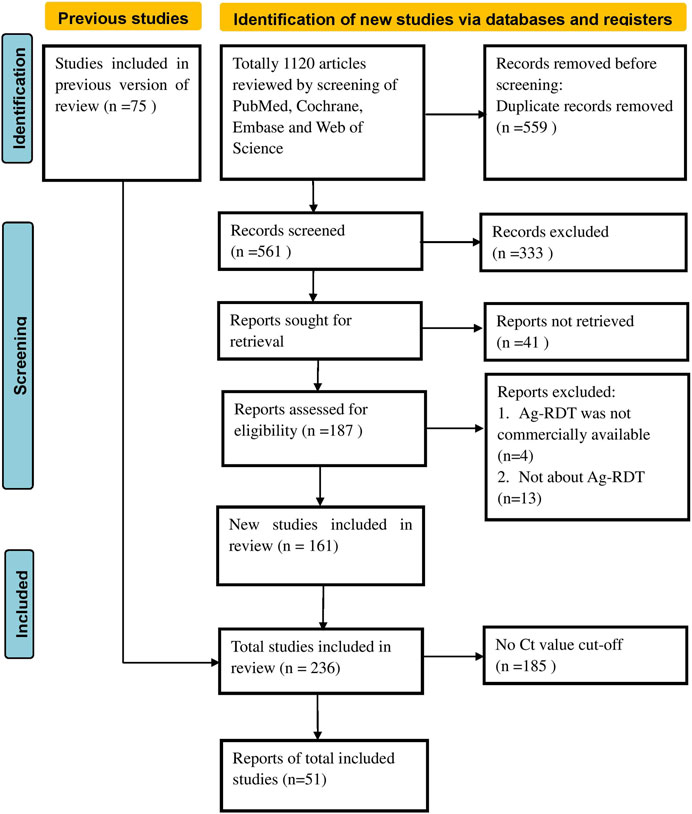
FIGURE 1. Preferred reporting items for systematic reviews and meta-analyses flow chart (Global, 2020–2023).
Afterward, data extraction was performed by an author per paper and reviewed by a second. The following data were extracted from included studies using a data-extraction form: first author, nation, sample size, sample condition, index tests, false positives, and Ct cut-off values.
Statistical Analysis
We used STATA Version 17.0 software to conduct the meta-analysis. Subgroup analysis was conducted based on different Ct value cut-offs (<40 or ≥40). The main indicators used were percentage ratios and 95% confidence intervals (CIs) of false positives in the RADTs group relative to those in the RT-PCR group. The I2 statistic was used to assess the level of statistical heterogeneity, and an I2 value of ≥50% confirmed heterogeneity [15]. We conducted a random-effect model meta-analysis because we expected considerable clinical heterogeneity. In addition to an overall evaluation, we also conducted a sub-group meta-analysis for Ct value cut-off with <40 and those ≥40. We evaluated the quality of the evidence for each outcome using the GRADE (Grading of Recommendations Assessment, Development and Evaluation) protocols, which classified evidence as very low, low, moderate, or high [16].
Results
The main characteristics of the included studies are summarized in Table 2.
All 51 included studies [17–67] evaluated the false positive rates of RADTs with respect to the standard RT- PCR while reporting a Ct cut-off value. The most common sample type evaluated was NP (44/51), followed by mixed NP/OP (5/51). We divided the 51 studies into 74 data sets. The overall pooled estimates of false positive rates of RADTs were 1% (95% CI: 0.00–0.01) compared to RT-PCR (Figure 2). In the stratified analysis, a similar pattern was observed when Ct-values were analyzed using cut-off <40 or ≥40, resulting in an estimated false positive rate of 1% (95% CI: 0.00–0.01) and 1% (95% CI: 0.00–0.01), respectively (Figures 3A,B).
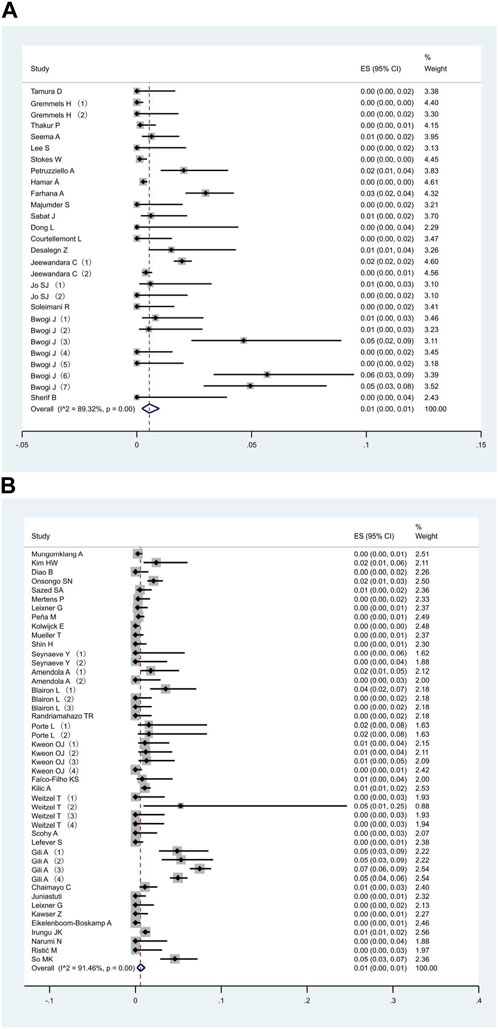
FIGURE 3. Meta-analysis of the false positive in people with Ct value cut-off <40 (A) and ≥40 (B) (Global, 2020–2023).
The summary of findings and the GRADE assessment for each outcome is presented in Table 3. The quality of evidence from the included studies was initially judged to be moderate due to imprecision.
Discussion
Clinical Implications
Although COVID-19 is not a chronic disease, it meets the Wilson criteria for screening due to the following facts: it is an important health problem; its natural history and side effects are well understood; there is a recognizable latent or early symptomatic stage; a test is easy to perform and its acceptably, accurately, reliably, sensitively and specifically easy to interpret; an accepted treatment is recognized for the disease; treatment is more effective if started early; a policy on who should be treated has been in execution; timely diagnosis and treatment are cost-effective, and case finding should be a continuous process [68].
To our knowledge, this is the first systematic review and meta-analysis that explores the relationship between RADTs and Ct value cut-offs. We integrated data from 51 studies to evaluate the false positive rates of RADTs. We found an overall satisfactory false positive rate (0.01, 95% CI: 0.00–0.01) for RADTs compared to RT-PCR. In the stratified analysis, we also found that false positive rates of RADTs did not increase when Ct values of RT-PCR increased (Ct < 40, 0.01, 95% CI: 0.00–0.01; Ct ≥ 40, 95% CI: 0.00–0.01).
Amplification of genomic sequence of RT-PCR is measured in Ct values. Reporting this Ct value or calculating viral load aids in interpretation and clinical decision-making [69]. However, there are controversies on how thresholds for infectivity shall be defined [70]. A previously published meta-analysis [71] showed that
RADTs present high sensitivity and specificity in detecting COVID-19 but not in exploring the relationship between Ct values and sensitivity/specificity. Since it is difficult to standardize Ct values across different systems and research teams, we could not compare the results between the studies included with different Ct values [71]. Many qPCR assays introduced a Ct cut-off of 40 for infectivity, allowing the detection of very few starting RNA molecules [69]. Our study was able to prove further the effectiveness of the association between RADTs and RT-PCR in different groups of Ct-Values.
Furthermore, it is of great necessity to carry out mass testing in communities as a major strategy for transmission control to minimize the spread of the infection because statistics show that those who never develop symptoms or are still in the before-symptom-onset stage can be quite contagious [72]. Therefore, self-testing using RADTs is vital in low-cost, resource-saving infection control [73]. Meanwhile, swab sampling from the oral or anterior nasal (AN) is less invasive and more efficient in improving compliance and testing frequency. Therefore, if such tests are adopted in community screening, especially in densely populated sites like schools and universities, rest homes, clinics, and prisons, they may help to reduce or even eliminate transmission significantly [58]. As it is easy to perform, all individuals can collect samples independently. This would eventually reduce the quarantine time for the potentially infected [69] and allow individuals to return to their regular work as early as possible. But the prerequisite for a reliable and validated SARS-CoV-2 test is compliance with negative and traditional physical preventive measures like face masks and social distancing that must be strictly observed [74]. Besides, such diagnostic capability would also enable policymakers to evaluate and adjust restrictive rules like quarantine duration and business shutdown period to ensure quick recovery of the economy.
The clinicians could also adjust discharge criteria according to Ct values and determine when the patient can discontinue isolation (which will shorten the hospitalization and isolation time of patients further and avoid unnecessary isolation treatment and waste of medical resources). The adjustment of Ct-values has implications in public health screening, enabling contact tracers to focus on persons who are most likely to be infectious. Reduced screening performance induced by the low sensitivity of RADTs makes it essential to guarantee effective repetition in close screening [75, 76].
Clinical Practice
The COVID-19 pandemic threatened the effective management of hospital risk. Health care providers should respond timely and effectively to rapidly changing regulations and guidelines [77]. During this period, hospitals need to care for COVID-19 patients, but also those with other diseases. The lower false positive rate and costs of RADTs imply that early and suitable detection was effective.
Nevertheless, the consequences of failing to identify and separate those COVID-19 cases affect the quality of care [78, 79]. Cases that were negative on the RADTs but had clinical symptoms were sent for further RT-PCR testing for timely identification of COVID-19 cases.
RT-PCR is the most important indicator for the clinical diagnosis of COVID-19. However, since it requires substantial manpower and time, rapid and accurate laboratory diagnosis technology is very important. The results of this study implied that RADTs could be considered an alternative for the rapid triage of patients. Although manufacturers’ instructions vary, our study provides guidance in the real world: RADTs are easy to conduct, do not require expert knowledge, and the results are obtained within a few minutes, saving time and money. If suspicious patients had similar clinical symptoms but were negative for RCTs, they were further tested with RT-PCR. In addition, RADTs are also suitable for epidemiological analysis, such as group epidemic monitoring and contact tracing. Such integrated strategies could significantly enhance prevention.
RADTs is not only a reasonably inexpensive, simple test with quick results and more accessibility to patients, but is also an important tool that might be more useful in cutting off the chain of transmission by rapidly identifying positive and previous cases, discovering vast numbers of asymptomatic carriers who often migrate from one location to another [80, 81]. In addition, RDTs can provide additional seroepidemiological data and aetiological diagnosis to determine the magnitude of COVID-19 spread within a population [80]. Thus, previous studies indicated that RADTs were recommended for the early detection of patients suspected of having COVID-19 at the peripheral level of the health system and outside hospital settings in low- and middle-income countries [80–82], where there is little access to molecular tests.
Heterogeneity of Meta-Analysis
In the meta-analysis, heterogeneity exists if the sample estimates for the population risk were of different magnitudes [83]. In this study, we used the random effect model when I2 statistics were 89.32%, 91.46%, and 91.02% for Ct values < 40, Ct values ≥ 40, and overall, respectively. Because of the existence of significant heterogeneity in false positive rates, it is important to assess heterogeneity in the meta-analysis. This could be caused by various factors, including population characteristics, study design, sample quality, antigen test manufacturers’ instructions, and Ct cut-off values [7]. We aggregated studies that explicitly reported a Ct cut-off value, but heterogeneity in the results was inevitable.
Methodological Considerations
The strengths of this study are as follows: First, we included all relevant studies from a global database, which are accepted with a relatively high level of evidence. Second, we excluded studies with no control groups to increase comparability and decrease possible heterogeneity. This is because a larger publication bias may exist without control groups. Third, a subgroup meta-analysis was performed to analyze the real association that controlled the independent Ct values. We analyzed the separate effects of the association between RADTs and RT-PCR based on different CT values.
However, there are still several limitations that should be noted when interpreting the findings of this meta-analysis. Firstly, because sources of reagents are very complex and no single diagnosis standard exists, the bias estimated is inevitable. Secondly, ordinary meta-analyses on efficacy render high-quality evidence from randomized controlled trials only. However, it is impossible to randomize people into “RADTs” and “RT-PCR” categories. Thirdly, few studies considered other potential confounding factors. Fourthly, the variable studied is only at Ct values in a rough range with a cut-off of <40 or ≥40, and it has failed to classify CT value cut-offs in more groups, such as below 29, 30–37, 38–40, and above 40, and add any other factors, for example the type of RADTs and RT-PCRs, the day the patient was examined, the type of reagent, the time to complete the examination, etc. Fifthly, when using the GRADE approach to evaluate the quality of the evidence for each outcome, the current evidence from all selected studies was moderate in imprecision. In the future, more comprehensive studies are recommended to improve the quality of evidence. Finally, only English papers were included, it was very difficult to explore the disparity of languages for the relationship between RADTs and RT-PCRs.
Conclusion
In conclusion, the best available evidence supports an association between RADTs and RT-PCR. When Ct-values were analyzed using cut-off <40 or ≥40, this resulted in an estimated false positive rate of only 1%. However, it is limited, and more trials are warranted. Further studies regarding subgroups according to sex and age are essential to clarifying the subgroup effect.
Author Contributions
T-HT conceived the study. Y-PY and ZJ collected the data. Y-PY, T-HT, and ZJ was responsible for the coding of the analyses. Y-PY wrote the first draft of the paper. Y-PY and ZJ searched, sorted and interpreted the relevant literature. All authors contributed to the article and approved the submitted version.
Conflict of Interest
The authors declare that they do not have any conflicts of interest.
References
1. Khandia, R, Singhal, S, and Alqahtani, T. Emergence of SARS-CoV-2 Omicron (B.1.1.529) Variant, Salient Features, High Global Health Concerns and Strategies to Counter it Amid Ongoing COVID-19 Pandemic. Environ Res (2022) 209:112816. doi:10.1016/j.envres.2022.112816
2. Planas, D, Saunders, N, and Maes, P. Considerable Escape of SARS-CoV-2 Omicron to Antibody Neutralization. Nature (2022) 602(7898):671–5. doi:10.1038/s41586-021-04389-z
3. Taylor, L. Covid-19: Omicron Drives Weekly Record High in Global Infections. BMJ (2022) 376:o66. doi:10.1136/bmj.o66
4. Contreras, S, Dehning, J, Loidolt, M, Zierenberg, J, Paul Spitzner, F, Jorge, H, et al. The Challenges of Containing SARS-CoV-2 via Test-Trace-And-Isolate. Nat Commun (2021) 12:378. doi:10.1038/s41467-020-20699-8
5. Li, Z, Yi, Y, Luo, X, Xiong, N, Liu, Y, Li, S, et al. Development and Clinical Application of a Rapid IgM-IgG Combined Antibody Test for SARS-CoV-2 Infection Diagnosis. J Med Virol (2020) 92(9):1518–24. doi:10.1002/jmv.25727
6. Ilkhani, H, Hedayat, N, and Farhad, S. Novel Approaches for Rapid Detection of COVID-19 During the Pandemic: A Review. Anal Biochem (2021) 634:114362. doi:10.1016/j.ab.2021.114362
7. Dinnes, J, Deeks, JJ, and Berhane, S. Rapid, Point-Of-Care Antigen and Molecular-Based Tests for Diagnosis of SARS-CoV-2 Infection. Cochrane Database Syst Rev (2021) 3(3):CD013705. doi:10.1002/14651858.CD013705.pub2
8. García-Fiñana, M, Hughes, DM, and Cheyne, CP. Performance of the Innova SARS-CoV-2 Antigen Rapid Lateral Flow Test in the Liverpool Asymptomatic Testing Pilot: Population Based Cohort Study. BMJ (2021) 374:n1637. doi:10.1136/bmj.n1637
9. Corman, VM, Landt, O, Kaiser, M, Molenkamp, R, Meijer, A, Chu, DK, et al. Detection of 2019 Novel Coronavirus (2019-nCoV) by Real-Time RT-PCR. Euro Surveill (2020) 25(3):2000045. doi:10.2807/1560-7917.ES.2020.25.3.2000045
10.World Health Organization. Antigen-Detection in the Diagnosis of SARS-CoV- 2 Infection: Interim Guidance (2021). Available from: https://apps.who.int/iris/handle/10665/345948 (Accessed December 8, 2021).
11. Aranaz-Andrés, JM, Chávez, ACF, and Laso, AM. Analysis of the Diagnostic Accuracy of Rapid Antigenic Tests for Detection of SARS-CoV-2 in Hospital Outbreak Situation. Eur J Clin Microbiol Infect Dis (2022) 41(2):305–12. doi:10.1007/s10096-021-04346-8
12. Peeling, RW, Olliaro, PL, and Boeras, DI. Scaling up COVID-19 Rapid Antigen Tests: Promises and Challenges. Lancet Infect Dis (2021) 21:e290–e295. doi:10.1016/S1473-3099(21)00048-7
13. Brümmer, LE, Katzenschlager, S, McGrath, S, Schmitz, S, Gaeddert, M, Erdmann, C, et al. Accuracy of Rapid Point-Of-Care Antigen-Based Diagnostics for SARS-CoV-2: An Updated Systematic Review and Meta-Analysis with Meta-Regression Analyzing Influencing Factors. Plos Med (2022) 19(5):e1004011. doi:10.1371/journal.pmed.1004011
14. Yang, YP, Huang, LL, Pan, SJ, Xu, D, Jiesisibieke, ZL, and Tung, TH. False-Positivity Results in Rapid Antigen Tests for SARS-CoV-2: An Umbrella Review of Meta-Analyses and Systematic Reviews. Expert Rev Anti Infect Ther (2022) 20(7):1005–13. doi:10.1080/14787210.2022.2070152
15. Higgins, JP, Thompson, SG, and Deeks, JJ. Measuring Inconsistency in Meta-Analyses. BMJ (2003) 327(7414):557–60. doi:10.1136/bmj.327.7414.557
16. Guyatt, GH, Oxman, AD, Vist, GE, Kunz, R, Falck-Ytter, Y, Alonso-Coello, P, et al. GRADE: An Emerging Consensus on Rating Quality of Evidence and Strength of Recommendations. BMJ (2008) 336(7650):924–6. doi:10.1136/bmj.39489.470347.AD
17. Tamura, D, Yamagishi, H, Morisawa, Y, Mato, T, Nunomiya, S, Maehara, Y, et al. Diagnostic Accuracy of a Novel SARS CoV-2 Rapid Antigen Test and Usefulness of Specimens Collected From the Anterior Nasal Cavity. Int J Infect Dis (2022) 124:199–205. doi:10.1016/j.ijid.2022.09.018
18. Gremmels, H, Winkel, BMF, Schuurman, R, Rosingh, A, Rigter, NAM, Rodriguez, O, et al. Real-Life Validation of the Panbio™ COVID-19 Antigen Rapid Test (Abbott) in Community-Dwelling Subjects with Symptoms of Potential SARS-CoV-2 Infection. EClinicalMedicine (2021) 31:100677. doi:10.1016/j.eclinm.2020.100677
19. Thakur, P, Saxena, S, Manchanda, V, Rana, N, Goel, R, and Arora, R. Utility of Antigen-Based Rapid Diagnostic Test for Detection of SARS-CoV-2 Virus in Routine Hospital Settings. Lab Med (2021) 52(6):e154–e158. doi:10.1093/labmed/lmab033
20. Seema, A, Naziya, Z, Asif, J, and Salim Khan, SM. Diagnostic Accuracy of STANDARD Q COVID-19 Antigen Detection Kit in Comparison with RT-PCR Assay Using Nasopharyngeal Samples in India. J Clin Diagn Res (2022) 16(1):DC01–DC05. doi:10.7860/JCDR/2022/52286.15825
21. Lee, S, Widyasari, K, Yang, HR, Jang, J, Kang, T, and Kim, S. Evaluation of the Diagnostic Accuracy of Nasal Cavity and Nasopharyngeal Swab Specimens for SARS-CoV-2 Detection via Rapid Antigen Test According to Specimen Collection Timing and Viral Load. Diagnostics (Basel) (2022) 12(3):710. doi:10.3390/diagnostics12030710
22. Stokes, W, Berenger, BM, Portnoy, D, Scott, B, Szelewicki, J, Singh, T, et al. Clinical Performance of the Abbott Panbio with Nasopharyngeal, Throat, and Saliva Swabs Among Symptomatic Individuals with COVID-19. Eur J Clin Microbiol Infect Dis (2021) 40(8):1721–6. doi:10.1007/s10096-021-04202-9
23. Petruzziello, A, Sabatino, R, Catapane, LA, De Falco, C, Petti, A, Tripaldelli, E, et al. Analytical Performance Evaluation of Lumipulse® SARS-CoV-2 Antigen Assay in 392 Asymptomatic Patients. J Clin Lab Anal (2023) 37(6):e24867. doi:10.1002/jcla.24867
24. Hamar, Á, Filipánits, K, Váradi, A, Váradi-Rácz, R, Gellén, HO, Futács, K, et al. Diagnostic Accuracy of SARS-CoV-2 Panbio™ Rapid Antigen Diagnostic Tests in a 4,440-Case Clinical Follow-Up. Front Med (Lausanne) (2022) 9:908127. doi:10.3389/fmed.2022.908127
25. Farhana, A, Zahoor, D, Wani, S, Khan, RA, Nasir, R, and Kanth, F. Diagnostic Utility and Performance of Rapid Antigen Test in SARS CoV- 2 in Symptomatic and Asymptomatic Patients During the Second Pandemic Wave in Kashmir, North India. Indian J Med Microbiol (2022) 40(4):572–6. doi:10.1016/j.ijmmb.2022.06.007
26. Majumder, S, Chakrabarti, A, Das, B, Sarkar, A, and Majumdar, T. Comparison of SARS-CoV-2 Diagnosis by Rapid Antigen Detection Kit with RT-qPCR in a Tertiary Care Setup in North Eastern India. Indian J Med Microbiol (2023) 42:12–6. doi:10.1016/j.ijmmb.2022.12.016
27. Sabat, J, Subhadra, S, Rath, S, Ho, LM, Satpathy, T, Pattnaik, D, et al. A Comparison of SARS-CoV-2 Rapid Antigen Testing with Realtime RT-PCR Among Symptomatic and Asymptomatic Individuals. BMC Infect Dis (2023) 23(1):87. doi:10.1186/s12879-022-07969-0
28. Dong, L, Li, WF, and Jiang, Y. Performance Evaluation of Antigen Detection Rapid Diagnostic Test (Ag-RDT) for COVID-19 Diagnosis in a Primary Healthcare Center During the Shanghai COVID-19 Quarantine Period. Virol J (2022) 19(1):140. doi:10.1186/s12985-022-01871-6
29. Courtellemont, L, Guinard, J, Guillaume, C, Giaché, S, Rzepecki, V, Seve, A, et al. High Performance of a Novel Antigen Detection Test on Nasopharyngeal Specimens for Diagnosing SARS-CoV-2 Infection. J Med Virol (2021) 93(5):3152–7. doi:10.1002/jmv.26896
30. Desalegn, Z, Sebre, S, Yohannes, M, Seman, A, Shiferaw, W, Ademe, M, et al. Comparison of the Diagnostic Performance of a Rapid Antigen Test with Real-Time Polymerase Chain Reaction for Detection of SARS-CoV-2 Among Patients Diagnosed with COVID-19 at Selected Hospitals in Addis Ababa, Ethiopia. Infect Drug Resist (2022) 15:4299–305. doi:10.2147/IDR.S353844
31. Jeewandara, C, Guruge, D, Pushpakumara, PD, Madhusanka, D, Jayadas, TT, Chaturanga, IP, et al. Sensitivity and Specificity of Two WHO Approved SARS-CoV2 Antigen Assays in Detecting Patients with SARS-CoV2 Infection. BMC Infect Dis (2022) 22(1):276. doi:10.1186/s12879-022-07240-6
32. Jo, SJ, Shin, SH, Kim, J, Lee, S, and Lee, J. Evaluation of the Clinical Performance of a Magnetic Force-Assisted Electrochemical Immunoassay for the Detection of SARS-CoV-2 Antigens. PLoS One (2021) 16(10):e0258394. doi:10.1371/journal.pone.0258394
33. Soleimani, R, Deckers, C, Huang, TD, Bogaerts, P, Evrard, S, Wallemme, I, et al. Rapid COVID-19 Antigenic Tests: Usefulness of a Modified Method for Diagnosis. J Med Virol (2021) 93(9):5655–9. doi:10.1002/jmv.27094
34. Bwogi, J, Lutalo, T, Tushabe, P, Bukenya, H, Eliku, JP, Ssewanyana, I, et al. Field Evaluation of the Performance of Seven Antigen Rapid Diagnostic Tests for the Diagnosis of SARs-CoV-2 Virus Infection in Uganda. PLoS One (2022) 17(5):e0265334. doi:10.1371/journal.pone.0265334
35. Sherif, B, Hafez, HM, Abdelhalim, MR, Elwafa, MAZA, Wahba, NS, and Hamdy, P. Evaluation of Diagnostic Performance of SARS-CoV-2 Detection Kits: a Comparative Study. Beni Suef Univ J Basic Appl Sci (2023) 12(1):17. doi:10.1186/s43088-023-00360-1
36. Mungomklang, A, Trichaisri, N, Jirachewee, J, Sukprasert, J, Tulalamba, W, and Viprakasit, V. Limited Sensitivity of a Rapid SARS-CoV-2 Antigen Detection Assay for Surveillance of Asymptomatic Individuals in Thailand. Am J Trop Med Hyg (2021) 105(6):1505–9. doi:10.4269/ajtmh.21-0809
37. Kim, HW, Park, M, and Lee, JH. Clinical Evaluation of the Rapid STANDARD Q COVID-19 Ag Test for the Screening of Severe Acute Respiratory Syndrome Coronavirus 2. Ann Lab Med (2022) 42(1):100–4. doi:10.3343/alm.2022.42.1.100
38. Diao, B, Wen, K, Zhang, J, Chen, J, Han, C, Chen, Y, et al. Accuracy of a Nucleocapsid Protein Antigen Rapid Test in the Diagnosis of SARS-CoV-2 Infection. Clin Microbiol Infect (2021) 27(2):289.e1–289.e4. doi:10.1016/j.cmi.2020.09.057
39. Onsongo, SN, Otieno, K, van Duijn, S, Adams, E, Omollo, M, Odero, IA, et al. Performance of a Rapid Antigen Test for SARS-CoV-2 in Kenya. Diagn Microbiol Infect Dis (2022) 102(2):115591. doi:10.1016/j.diagmicrobio.2021.115591
40. Sazed, SA, Kibria, MG, Hossain, MS, Zamil, MF, Adhikary, PC, Ahmed, D, et al. Clinical Evaluation of a New Antigen-Based COVID-19 Rapid Diagnostic Test From Symptomatic Patients. Diagnostics (Basel) (2021) 11(12):2300. doi:10.3390/diagnostics11122300
41. Mertens, P, De Vos, N, Martiny, D, Jassoy, C, Mirazimi, A, Cuypers, L, et al. Development and Potential Usefulness of the COVID-19 Ag Respi-Strip Diagnostic Assay in a Pandemic Context. Front Med (Lausanne) (2020) 7:225. doi:10.3389/fmed.2020.00225
42. Leixner, G, Voill-Glaninger, A, Bonner, E, Kreil, A, Zadnikar, R, and Viveiros, A. Evaluation of the AMP SARS-CoV-2 Rapid Antigen Test in a Hospital Setting. Int J Infect Dis (2021) 108:353–6. doi:10.1016/j.ijid.2021.05.063
43. Peña, M, Ampuero, M, Garcés, C, Gaggero, A, García, P, Velasquez, MS, et al. Performance of SARS-CoV-2 Rapid Antigen Test Compared with Real-Time RT-PCR in Asymptomatic Individuals. Int J Infect Dis (2021) 107:201–4. doi:10.1016/j.ijid.2021.04.087
44. Kolwijck, E, Brouwers-Boers, M, Broertjes, J, van Heeswijk, K, Runderkamp, N, Meijer, A, et al. Validation and Implementation of the Panbio COVID-19 Ag Rapid Test for the Diagnosis of SARS-CoV-2 Infection in Symptomatic Hospital Healthcare Workers. Infect Prev Pract (2021) 3(2):100142. doi:10.1016/j.infpip.2021.100142
45. Mueller, T, Kompatscher, J, and La Guardia, M. Diagnostic Performance of the Elecsys SARS-CoV-2 Antigen Assay in the Clinical Routine of a Tertiary Care Hospital: Preliminary Results From a Single-Center Evaluation. J Clin Lab Anal (2021) 35(8):e23906. doi:10.1002/jcla.23906
46. Shin, H, Lee, S, Widyasari, K, Yi, J, Bae, E, and Kim, S. Performance Evaluation of STANDARD Q COVID-19 Ag Home Test for the Diagnosis of COVID-19 During Early Symptom Onset. J Clin Lab Anal (2022) 36(6):e24410. doi:10.1002/jcla.24410
47. Seynaeve, Y, Heylen, J, Fontaine, C, Maclot, F, Meex, C, Diep, AN, et al. Evaluation of Two Rapid Antigenic Tests for the Detection of SARS-CoV-2 in Nasopharyngeal Swabs. J Clin Med (2021) 10(13):2774. doi:10.3390/jcm10132774
48. Amendola, A, Sberna, G, Lalle, E, Colavita, F, Castilletti, C, Menchinelli, G, et al. Saliva is a Valid Alternative to Nasopharyngeal Swab in Chemiluminescence-Based Assay for Detection of SARS-CoV-2 Antigen. J Clin Med (2021) 10(7):1471. doi:10.3390/jcm10071471
49. Blairon, L, Cupaiolo, R, Thomas, I, Piteüs, S, Wilmet, A, Beukinga, I, et al. Efficacy Comparison of Three Rapid Antigen Tests for SARS-CoV-2 and How Viral Load Impact Their Performance. J Med Virol (2021) 93(10):5783–8. doi:10.1002/jmv.27108
50. Randriamahazo, TR, Andrianarivelo, AM, Rakotoarivo, AT, Raheritiana, TM, Rakotovao, LA, Randriamanantany, ZA, et al. Evaluation of Antigen-Based Rapid Detection Test for the Diagnosis of SARS CoV-2 in Low-Income Countries. J Virol Methods (2022) 300:114409. doi:10.1016/j.jviromet.2021.114409
51. Porte, L, Legarraga, P, Iruretagoyena, M, Vollrath, V, Pizarro, G, Munita, J, et al. Evaluation of Two Fluorescence Immunoassays for the Rapid Detection of SARS-CoV-2 Antigen-New Tool to Detect Infective COVID-19 Patients. PeerJ (2021) 9:e10801. doi:10.7717/peerj.10801
52. Kweon, OJ, Lim, YK, Kim, HR, Choi, Y, Choi, SH, Chung, JW, et al. Evaluation of Rapid SARS-CoV-2 Antigen Tests, AFIAS COVID-19 Ag and Ichroma COVID-19 Ag, with Serial Nasopharyngeal Specimens From COVID-19 Patients. PLoS One (2021) 16(4):e0249972. doi:10.1371/journal.pone.0249972
53. Faíco-Filho, KS, Júnior, FEF, Moreira, LVL, Lins, PRG, Justo, AFO, and Bellei, N. Evaluation of the Panbio™ COVID-19 Ag Rapid Test at an Emergency Room in a Hospital in São Paulo, Brazil. Braz J Infect Dis (2022) 26(2):102349. doi:10.1016/j.bjid.2022.102349
54. Kilic, A, Hiestand, B, and Palavecino, E. Evaluation of Performance of the BD Veritor SARS-CoV-2 Chromatographic Immunoassay Test in Patients with Symptoms of COVID-19. J Clin Microbiol (2021) 59(5):e00260-21. doi:10.1128/JCM.00260-21
55. Weitzel, T, Legarraga, P, Iruretagoyena, M, Pizarro, G, Vollrath, V, Araos, R, et al. Comparative Evaluation of Four Rapid SARS-CoV-2 Antigen Detection Tests Using Universal Transport Medium. Trav Med Infect Dis (2021) 39:101942. doi:10.1016/j.tmaid.2020.101942
56. Scohy, A, Anantharajah, A, and Bodéus, M. Low Performance of Rapid Antigen Detection Test as Frontline Testing for COVID-19 Diagnosis. J Clin Virol (2020) 129:104455. doi:10.1016/j.jcv.2020.104455
57. Lefever, S, Indevuyst, C, Cuypers, L, Dewaele, K, Yin, N, Cotton, F, et al. Comparison of the Quantitative DiaSorin Liaison Antigen Test to Reverse Transcription-PCR for the Diagnosis of COVID-19 in Symptomatic and Asymptomatic Outpatients. J Clin Microbiol (2021) 59(7):e0037421. doi:10.1128/JCM.00374-21
58. Gili, A, Paggi, R, Russo, C, Cenci, E, Pietrella, D, Graziani, A, et al. Evaluation of Lumipulse® G SARS-CoV-2 Antigen Assay Automated Test for Detecting SARS-CoV-2 Nucleocapsid Protein (NP) in Nasopharyngeal Swabs for Community and Population Screening. Int J Infect Dis (2021) 105:391–6. doi:10.1016/j.ijid.2021.02.098
59. Chaimayo, C, Kaewnaphan, B, and Tanlieng, N. Rapid SARS-CoV-2 Antigen Detection Assay in Comparison with Real-Time RT-PCR Assay for Laboratory Diagnosis of COVID-19 in Thailand. Virol J (2020) 17(1):177. doi:10.1186/s12985-020-01452-5
60. Juniastuti, , Furqoni, AH, Amin, M, Restifan, YD, Putri, SMD, Ferandra, VA, et al. The Evaluation Results of Proposed Antigen Rapid Diagnostic Tests for COVID-19: Some Possible Factors Might Influence. Infection (2023) 2023:1–7. doi:10.1007/s15010-022-01975-9
61. Leixner, G, Voill-Glaninger, A, Krejci, I, Gaugeler-Kurzweil, J, Kusstatscher, T, Krugluger, W, et al. Performance Study of the Anterior Nasal AMP SARS-CoV-2 Rapid Antigen Test in Comparison with Nasopharyngeal rRT-PCR. Access Microbiol (2022) 4(6):acmi000361. doi:10.1099/acmi.0.000361
62. Kawser, Z, Hossain, M, Suliman, S, Lockman, S, Gitaka, J, Bandawe, G, et al. An Assessment of a Rapid SARS-CoV-2 Antigen Test in Bangladesh. Am J Trop Med Hyg (2022) 107(4):845–9. doi:10.4269/ajtmh.22-0068
63. Eikelenboom-Boskamp, A, den Ouden, M, de Groot, T, Stobernack, T, Wertheim, H, and Voss, A. Evaluation of the Abbott Panbio™ COVID-19 Antigen Detection Rapid Diagnostic Test Among Healthcare Workers in Elderly Care. PLoS One (2023) 18(2):e0276244. doi:10.1371/journal.pone.0276244
64. Irungu, JK, Munyua, P, Ochieng, C, Juma, B, Amoth, P, Kuria, F, et al. Diagnostic Accuracy of the Panbio COVID-19 Antigen Rapid Test Device for SARS-CoV-2 Detection in Kenya, 2021: A Field Evaluation. PLoS One (2023) 18(1):e0277657. doi:10.1371/journal.pone.0277657
65. Narumi, N, Kondo, T, Sato, Y, Katayama, Y, Nirasawa, S, Saeki, M, et al. Analysis of Diagnostic Performance and Factors Causing Nonspecific Reactions in SARS-CoV-2 Rapid Antigen Detection Tests. J Infect Chemother (2023) 29(2):157–62. doi:10.1016/j.jiac.2022.10.007
66. Ristić, M, Nikolić, N, Čabarkapa, V, Turkulov, V, and Petrović, V. Validation of the STANDARD Q COVID-19 Antigen Test in Vojvodina, Serbia. PLoS One (2021) 16(2):e0247606. doi:10.1371/journal.pone.0247606
67. So, MK, Chung, HS, Lee, DH, and Lee, M. How Significant are Xpert Xpress SARS-CoV-2 Test Findings When Only an N2 Gene is Detected? Diagnostics (Basel) (2022) 12(9):2133. doi:10.3390/diagnostics12092133
68. Shen, HC, Hu, YC, Chen, YF, and Tung, TH. Prevalence and Associated Metabolic Factors of Gallstone Disease in the Elderly Agricultural and Fishing Population of Taiwan. Gastroenterol Res Pract (2014) 2014:876918. doi:10.1155/2014/876918
69. Tom, MR, and Mina, MJ. To Interpret the SARS-CoV-2 Test, Consider the Cycle Threshold Value. Clin Infect Dis (2020) 71:2252–4. doi:10.1093/cid/ciaa619
70. Romero-Gómez, MP, Gómez-Sebastian, S, Cendejas-Bueno, E, Montero-Vega, MD, Mingorance, J, and García-Rodríguez, JSARS-CoV-2 Working Group. Ct Value is not Enough to Discriminate Patients Harbouring Infective Virus. J Infect (2021) 82:e35–e37. doi:10.1016/j.jinf.2020.11.025
71. Fujita-Rohwerder, N, Beckmann, L, Zens, Y, and Verma, A. Diagnostic Accuracy of Rapid Point-Of-Care Tests for Diagnosis of Current SARS-CoV-2 Infections in Children: A Systematic Review and Meta-Analysis. BMJ Evid Based Med (2022) 27:274–87. doi:10.1136/bmjebm-2021-111828
72. Huff, HV, and Singh, A. Asymptomatic Transmission During the Coronavirus Disease 2019 Pandemic and Implications for Public Health Strategies. Clin Infect Dis (2020) 71:2752–6. doi:10.1093/cid/ciaa654
73. Ahmed, N, Kalil, MNA, Yusof, W, Bakar, MAA, Sjahid, AS, Hassan, R, et al. A Performance Assessment Study of Different Clinical Samples for Rapid COVID-19 Antigen Diagnosis Tests. Diagnostics (Basel) (2022) 12(4):847. doi:10.3390/diagnostics12040847
74. Mayor, S. Covid-19: Warning Over Transmission Risk as Self-Isolation is cut to Five Days in England. BMJ (2022) 376:o111. doi:10.1136/bmj.o111
75. Mina, JM, Parker, R, and Larremore, BD. Rethinking Covid-19 Test Sensitivity - a Strategy for Containment. N Engl J Med (2020) 383(22):e120. doi:10.1056/NEJMp2025631
76. Larremore, DB, Wilder, B, Lester, E, Shehata, S, Burke, JM, Hay, JA, et al. Test Sensitivity is Secondary to Frequency and Turnaround Time for COVID-19 Surveillance. Sci Adv (2020) 7(1):2020.06.22.20136309. doi:10.1101/2020.06.22.20136309
77. Tolentino, VR, Derevlany, L, DeLaMothe, C, Vick, S, and Chalyavski, L. The Effects of the COVID-19 Pandemic on Risk Management Practice: A Report From the Epicenter of the Epicenter in New York City. J Healthc Risk Manag (2021) 40(4):46–57. doi:10.1002/jhrm.21461
78. Wu, X, Zhou, H, and Wu, X. Strategies for Qualified Triage Stations and Fever Clinics During the Outbreak of COVID-2019 in the County Hospitals of Western Chongqing. J Hosp Infect (2020) 105(2):128–9. doi:10.1016/j.jhin.2020.03.021
79. Yu, X, and Yang, R. COVID-19 Transmission Through Asymptomatic Carriers is a challenge to Containment. Influenza Other Respir Viruses (2020) 14(4):474–5. doi:10.1111/irv.12743
80. Olalekan, A, Iwalokun, B, Akinloye, OM, Popoola, O, Samuel, TA, and Akinloye, O. COVID-19 Rapid Diagnostic Test Could Contain Transmission in Low- and Middle-Income Countries. Afr J Lab Med (2020) 9(1):1255. doi:10.4102/ajlm.v9i1.1255
81. Arshadi, M, Fardsanei, F, Deihim, B, Farshadzadeh, Z, Nikkhahi, F, Khalili, F, et al. Diagnostic Accuracy of Rapid Antigen Tests for COVID-19 Detection: A Systematic Review with Meta-Analysis. Front Med (Lausanne) (2022) 9:870738. doi:10.3389/fmed.2022.870738
82. Khandker, SS, Nik Hashim, NHH, Deris, ZZ, Shueb, RH, and Islam, MA. Diagnostic Accuracy of Rapid Antigen Test Kits for Detecting SARS-CoV-2: A Systematic Review and Meta-Analysis of 17,171 Suspected COVID-19 Patients. J Clin Med (2021) 10(16):3493. doi:10.3390/jcm10163493
Keywords: cycle threshold, false-positive, meta-analyses, rapid antigen test, RT-PCR
Citation: Yang Y-P, Jiesisibieke ZL and Tung T-H (2023) Association Between Rapid Antigen Detection Tests and Real-Time Reverse Transcription–Polymerase Chain Reaction Assay for SARS-CoV-2: A Systematic Review and Meta-Analyses. Int J Public Health 68:1605452. doi: 10.3389/ijph.2023.1605452
Received: 03 October 2022; Accepted: 11 July 2023;
Published: 01 August 2023.
Edited by:
Olaf von dem Knesebeck, University Medical Center Hamburg-Eppendorf, GermanyReviewed by:
Bijit Biswas, All India Institute of Medical Sciences, Deoghar (AIIMS Deoghar), IndiaEduardo Becerril, National Institute of Respiratory Diseases-Mexico (INER), Mexico
Copyright © 2023 Yang, Jiesisibieke and Tung. This is an open-access article distributed under the terms of the Creative Commons Attribution License (CC BY). The use, distribution or reproduction in other forums is permitted, provided the original author(s) and the copyright owner(s) are credited and that the original publication in this journal is cited, in accordance with accepted academic practice. No use, distribution or reproduction is permitted which does not comply with these terms.
*Correspondence: Tao-Hsin Tung, Y2gyODc2QGdtYWlsLmNvbQ==